Source |
Qian Zhang, Jiaxing Wang, Yanjun Ding, et al. Inhibition of Breast Cancer Cells by Novel Nanoparticles Combined with Low-Intensity Focused Ultrasound. Chinese Journal of Medical Imaging, 2020, 28(12): 907-911+917
|
Abstract |
Objective To explore the ability of the phase-change nanoparticles (Ce6@PFP/PLGA NPs) loaded with the sonosensitizer Chlorin e6 (Ce6) and perfluoropentane (PFP) combined with low-intensity focused ultrasound (LIFU) to enhance ultrasound imaging, promote apoptosis of breast cancer cells, and inhibit metastasis.
Materials and Methods The nanoparticles were prepared using a double-emulsion method, and their phase change and in vitro imaging were observed under different power levels of LIFU, and their safety was verified. The generation of reactive oxygen species was detected using a 2,7-dichlorodihydrofluorescein diacetate (DCFH-DA) fluorescence probe. The photodynamic therapy effect was verified using the Calcein/PI double staining method, and the inhibitory effect on the metastasis of 4T1 breast cancer cells was tested.
Results The Ce6@PFP/PLGA nanoparticles showed regular morphology under the microscope, with a particle size of (230.0±50.5) nm. After LIFU irradiation, the phase change and imaging effect were optimal at 4 W. After co-culturing the nanoparticles with 4T1 breast cancer cells, the cell survival rate was >85%; after the combined action of LIFU and the nanoparticles, apoptosis significantly increased, and cell migration was significantly inhibited, with migration areas of (187.72±4.02), (129.90±11.22), (120.83±10.47), and (31.87±1.16) square pixels, showing a statistically significant difference (F=131, P<0.05).
Conclusion The Ce6@PFP/PLGA nanoparticles play an important role in ultrasound imaging, photodynamic therapy, and inhibiting tumor cell metastasis under LIFU irradiation, and are expected to become a new model for breast cancer treatment.
【Keywords】Breast tumor; Tumor, experimental; Ultrasound, high-intensity focused; Sonodynamic therapy; Nanoparticles; In vitro study; Tumor metastasis
【Classification Number】R445.1;R737.9
【DOI】10.3969/j.issn.1005-5185.2020.12.007
|
Breast cancer is a serious threat to women’s health[1], and current conventional treatments include surgical resection, radiotherapy, chemotherapy, endocrine therapy, and targeted therapy, making the development of emerging treatment methods of great significance. The development of nanomedicine and precision therapy has opened new avenues for overcoming the challenges of breast cancer treatment, utilizing the unique properties of nanomaterials to achieve real-time dynamic imaging and drug delivery strategies targeting tumor areas. Sonodynamic therapy (SDT), developed based on ultrasound characteristics, has advantages such as non-invasiveness, no radiation, and simplicity, killing tumor cells by generating reactive oxygen species (ROS) and possessing unique cavitation effects of ultrasound, indicating broad therapeutic prospects. This study utilizes the newly constructed polymer composite nanoparticles Ce6@PFP/PLGA, mediated by low-intensity focused ultrasound (LIFU), to observe their inhibitory effects on the growth and metastasis of 4T1 breast cancer cells, exploring the feasibility of this as a precision treatment method for breast cancer and inhibiting metastasis.
1.1 Reagents and Instruments
PLGA-COOH (molecular weight 12,000 D, Jinan Daigang), perfluoropentane (perfluoropentane, PFP), agarose gel powder (Sigma), Chlorin e6 (Chlorin e6, Dalian Meilun), dichloromethane, methanol, isopropanol are all commercially available analytical grade products, polyvinyl alcohol (PVA, Shanghai Aladdin), CCK-8 reagent kit (Japan Tongren), 2,7-dichlorodihydrofluorescein diacetate (DCFH-DA) cell reactive oxygen species probe (USA MCE), Calcein-AM live cell dye (Calcein-AM, USA MCE), propidium iodide solution (PI, Beijing Soleibao), 4T1 cell line (Shanghai Cell Bank, Chinese Academy of Sciences), 650Y ultrasound vibrating instrument (Shanghai ZOLLO), laser particle size analyzer (Zetasizer Nano ZS-90, Malvern), Philips EPIQ5 ultrasound diagnostic instrument, low-intensity focused ultrasound therapy device (Chongqing Haifu Technology Co., Ltd.), laser confocal microscope (Olympus), Synergy multifunctional enzyme labeler (BioTek), low-temperature centrifuge (Eppendorf), UH 5300 ultraviolet spectrophotometer (HITACHI).
1.2 Preparation of Ce6@PFP/PLGA Nanoparticles
Weigh Ce6 2 mg, PLGA-COOH 50 mg. Dissolve Ce6 in methanol (500 μl) and mix with PLGA-COOH in 4 ml dichloromethane, adding 400 μl PFP, and sonicate under ice bath conditions for 3 min (duty cycle: 5 s∶5 s) to obtain the primary emulsion. Add 8 ml of 4% PVA solution and continue sonication for 3 min, obtaining the secondary emulsion. Add 10 ml 2% isopropanol dropwise, stir at room temperature for 4 h, then centrifuge at low temperature (12,000 r/min, 5 min) and wash three times to obtain the CPP nanoparticles, which are stored at 4℃ for later use.
1.3 Basic Characterization of Ce6@PFP/PLGA Nanoparticles
The size of the CPP nanoparticles was measured using a Malvern laser particle size analyzer, observing their normal optical morphology and transmission electron microscopy morphology; ultraviolet spectrophotometry was used to measure the UV absorption peaks of Ce6, PFP/PLGA, and different concentrations of Ce6@PFP/PLGA; a certain mass of Ce6 was diluted with methanol, and its absorbance was measured to create a standard curve to calculate the encapsulation rate of Ce6.
1.4 Detection of Phase Transition and Ultrasound Imaging Ability of Ce6@PFP/PLGA Nanoparticles
Dilute the previously prepared Ce6@PFP/PLGA nanoparticles to 1 mg/ml. Use the LIFU device for irradiation under different power conditions (2~5 W) for 60 s, then observe their phase change under the microscope; weigh 15 g of agarose powder and 400 ml of double-distilled water, heat in the microwave until dissolved, and stir until no bubbles are produced. Pour the liquid into a 2 ml centrifuge tube box and place it in a 2 ml centrifuge tube to solidify, obtaining the agarose gel model. Add 1 mg/ml CPP nanoparticles into the model holes, apply LIFU, and use the EPIQ 5 ultrasound diagnostic instrument (probe frequency 12 MHz, MI: 0.06) to observe different imaging results under conventional ultrasound and contrast-enhanced ultrasound (CEUS) modes.
1.5 Detection of Intracellular Reactive Oxygen Species Generation of Ce6@PFP/PLGA Nanoparticles
The intracellular ROS generation was detected using the DCFH-DA reactive oxygen species probe. After reacting with ROS, DCFH-DA can be converted into dichlorofluorescein (DCF), producing fluorescence used to evaluate the generation capacity of ROS under the action of LIFU in the CPP nanoparticles. The routinely cultured 4T1 breast cancer cells were divided into control group, LIFU group, CPP nanoparticles group, Ce6+LIFU group, and CPP nanoparticles + LIFU group, and cultured in a confocal culture dish for 24 h. Subsequently, according to different groups, add CPP nanoparticles or perform LIFU treatment. Add 100 μl diluted DCFH-DA solution for light-protected incubation for 15 min, then observe the intracellular ROS generation under the confocal microscope.
1.6 In Vitro Safety Testing of Ce6@PFP/PLGA Nanoparticles
Seed 4T1 cells at 1×104 cells/well in a 96-well plate and culture routinely for 24 h. Dilute the nanoparticles using serum-free 1640 culture medium, set different concentrations (0~0.6 mg/ml, n=5), and co-cultivate with cells for 6~24 h, then detect cell viability using the CCK-8 method.
1.7 In Vitro Experiments of LIFU Combined with Ce6@PFP/PLGA Nanoparticles for Enhanced Sonodynamic Therapy and Inhibition of Breast Cancer Cell Metastasis
1.7.1 Sonodynamic Therapy
Cultivate 4T1 cells in vitro, take logarithmic growth phase cells, and seed at 1×105 cells/ml in a confocal dish for 24 h and divide into 4 groups: control group, no treatment; LIFU group, cells only undergo LIFU irradiation (4 W, 60 s); CPP nanoparticles group, cells only add CPP nanoparticles; CPP nanoparticles + LIFU group, cells add CPP nanoparticles and then undergo LIFU irradiation (4 W, 60 s). Add 100 μl diluted Calcein-AM/PI solution, incubate in the dark for 20 min, then observe cell staining under the confocal microscope.
1.7.2 Inhibition of Breast Cancer Cell Metastasis
Seed 4T1 cells in the above groups into a 6 well plate. After regular adhesion, use a 10 μl pipette tip to make uniform scratches along the center of the 6-well plate in a sterile environment, and perform co-cultivation with nanoparticles or ultrasound treatment according to the groups, then continue to culture for 24 h, and observe cell migration under an optical microscope.
Use SPSS 22.0 software, with normally distributed measurement data expressed as ±s. Comparisons of migration areas among multiple groups were performed using one-way ANOVA, and pairwise comparisons were performed using the LSD method. P<0.05 indicates a statistically significant difference.
2.1 Basic Characteristics of Ce6@PFP/PLGA Nanoparticles
The prepared CPP nanoparticle aqueous solution is light gray, with uniform size and good dispersion under the microscope (Figure 1A), showing spherical shape under transmission electron microscopy (Figure 1B). The particle size of CPP nanoparticles measured by Malvern is (230.0±50.5) nm, with a dispersion of 0.048 (Figure 1C). The UV absorption spectra of Ce6, PFP/PLGA, and Ce6@PFP/PLGA suggest that Ce6 is successfully encapsulated in the CPP nanoparticles, with the UV spectrum of Ce6 showing a maximum absorption peak at 403 nm, which gradually increases with the concentration of CPP nanoparticles (Figure 1D), and the encapsulation rate of CPP was measured to be (91.11±2.25).
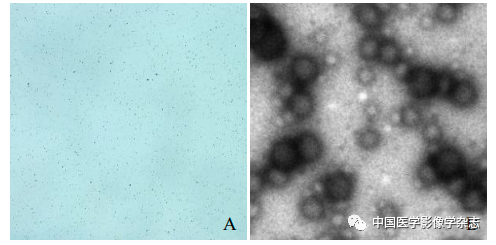
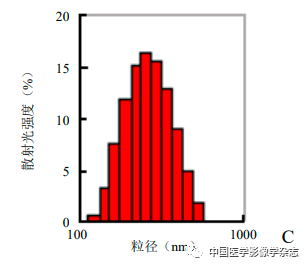
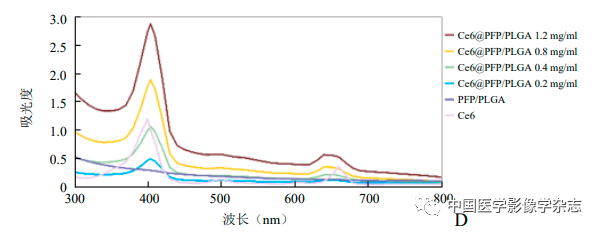
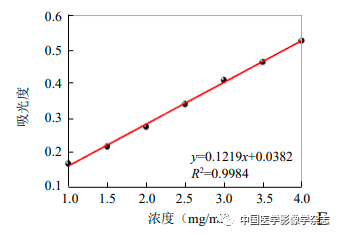
Figure1 Optical microscope (A) and transmission electron microscope (B) showing that Ce6@PFP/PLGA nanoparticles are stable and spherical; Ce6@PFP/PLGA nanoparticles have a relatively uniform particle size distribution (C); different concentrations of Ce6@PFP/PLGA nanoparticles and PFP/PLGA, Ce6 UV absorption spectra suggest effective encapsulation of Ce6 (D); based on the absorbance calculated standard curve of Ce6 at different concentrations (E)
2.2 Phase Transition Ability and Ultrasound Imaging Ability of Ce6@PFP/PLGA Nanoparticles
The CPP nanoparticles exhibited different phase transition effects under different ultrasound power irradiation. The results showed that 4 W irradiation for 60 s resulted in a more significant phase transition than other power levels (Figure 2A~D); the agarose gel model in 2D mode and imaging mode both showed the best imaging effect at 4 W (Figure 2E~H). Thus, the CPP nanoparticles successfully encapsulated the phase-change material PFP, and exhibited optimal ultrasound imaging functionality under 4 W LIFU irradiation.
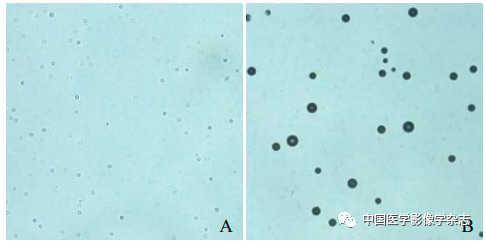
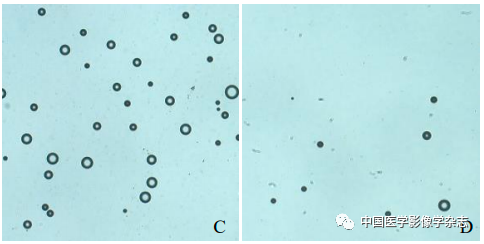
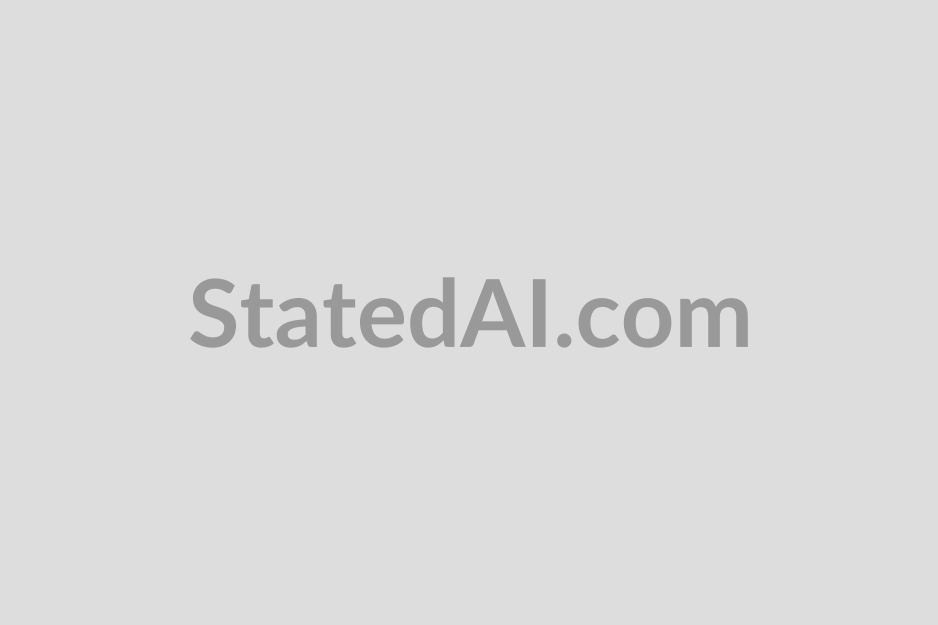
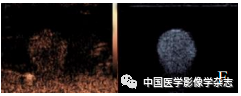
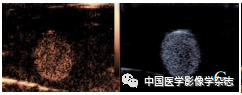
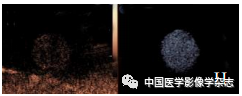
Figure2 The CPP nanoparticles exhibited different phase transition abilities after irradiation with different powers of LIFU. At 2 W, the nanoparticles slightly increased (A); at 3 W, bubbles began to appear (B); at 4 W, bubbles significantly increased (C); at 5 W, bubbles gradually decreased and disappeared; scale bar = 50 μm (D); the imaging results in both 2D and CEUS modes (2~5 W) are consistent with the phase transition results (E~H)
2.3 Intracellular ROS Generation Ability of Ce6@PFP/PLGA Nanoparticles
The confocal microscope showed that the CPP nanoparticles + LIFU group exhibited the most obvious green fluorescence, while the other groups showed no or only a small amount of green fluorescence (Figure 3), indicating that under the action of LIFU, the CPP nanoparticles have good ROS generation capacity.
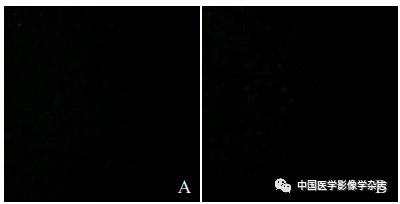
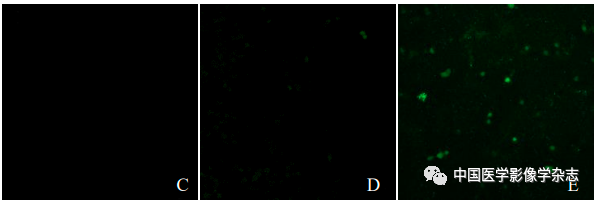
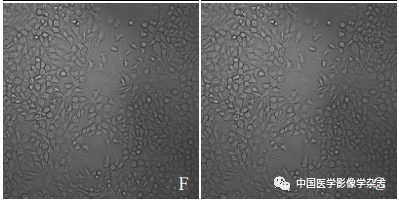
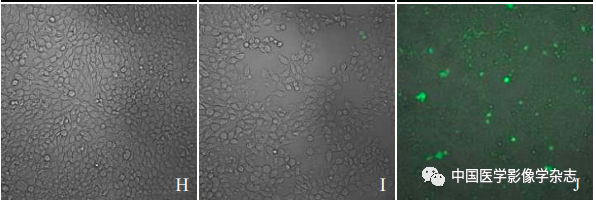
Figure3 The generation of ROS after different groups acted on 4T1 cells under the laser confocal microscope. A~E represent the control group, LIFU group, CPP nanoparticles group, Ce6+LIFU group, and CPP nanoparticles + LIFU group; F~J are the fusion results of confocal microscopy and optical microscopy for the above groups; scale bar = 50 μm
2.4 Cell Viability Detection of Ce6@PFP/PLGA Nanoparticles
The cell viability after co-culturing with CPP nanoparticles at different times and concentrations was determined using the CCK-8 method, showing that as the concentration of nanoparticles increased and the co-culturing time extended, cell viability remained above 85% (Figure 4), further proving the biocompatibility of CPP nanoparticles.
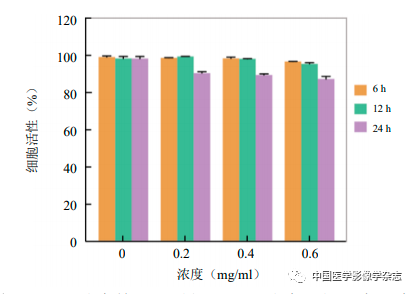
Figure4 The cell survival rate of CPP nanoparticles under different times and concentrations
2.5 In Vitro Experiments of LIFU Combined with Ce6@PFP/PLGA Nanoparticles for Enhanced SDT and Inhibition of Breast Cancer Metastasis
The laser confocal microscope showed that the CPP nanoparticles combined with LIFU (4 W) for 60 s resulted in the highest number of dead cells (Figure 5A); the other groups showed no significant number of cell death (Figure 5B~D). This indicates that the CPP nanoparticles under LIFU irradiation have good sonodynamic therapy effects.
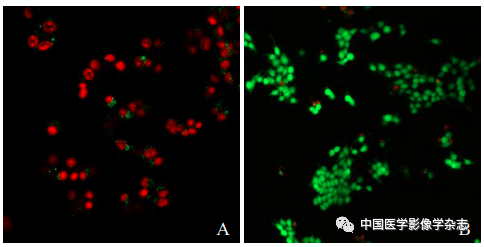
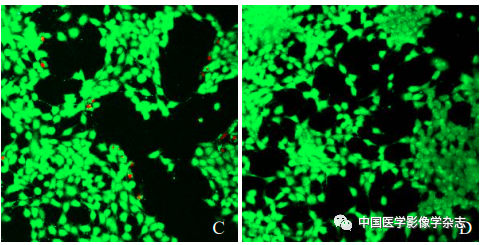
Figure5 The results of laser confocal microscopy for LIFU combined with Ce6@PFP/PLGA nanoparticles for SDT. A~D are respectively the CPP nanoparticles + LIFU group, LIFU group, CPP nanoparticles group, and control group; scale bar = 50 μm
2.5.2 Inhibition of Breast Cancer Cell Metastasis
The cell scratch experiment results showed that the CPP nanoparticles + LIFU group had the least cell migration after 24 h, with the largest migration area, and the differences compared to the other 3 groups were statistically significant (F=131.0, P<0.05, Figure 6), indicating that the LIFU combined with CPP nanoparticles SDT has the ability to inhibit the metastasis of 4T1 breast cancer cells.
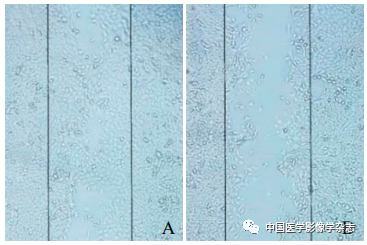
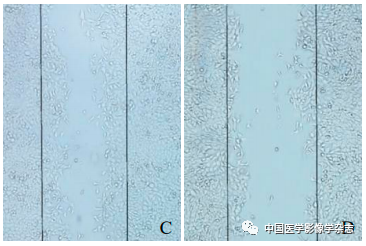
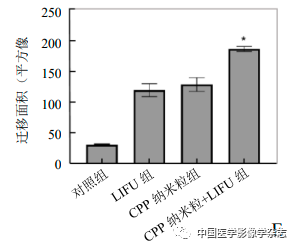
Figure6 The results of the cell scratch experiment. A~D represent the control group, LIFU group, CPP nanoparticles group, and CPP nanoparticles + LIFU group under optical microscope after 24 h; E shows the comparison between the CPP nanoparticles + LIFU group and the other 3 groups, *P<0.05
In recent years, SDT has attracted wide attention in the field of cancer treatment, utilizing ultrasound to activate sonosensitizers to produce ROS, damaging tumor cell DNA and triggering mitochondria-related tumor cell apoptosis, with unique deep penetration and no radiation damage advantages[2]; simultaneously, under various imaging guidance, SDT can also achieve precise treatment targeting tumor areas, contributing to the integrated development of tumor diagnosis and treatment[3]. Previously, ultrasound imaging was mostly achieved through microbubbles; however, microbubbles, due to their micron-sized nature, find it difficult to effectively accumulate in the lesion area using the tumor’s high permeability and retention effect. Additionally, the poor stability of microbubbles limits their application in targeted tumor therapy due to difficulties in modification and drug loading. The development of novel nanomaterials has achieved targeted tumor therapy while completing real-time dynamic imaging of tumors, which is of great significance for the diagnosis and treatment of various cancers[4].
This study utilized PLGA as the outer material to co-encapsulate PFP and Ce6, preparing multifunctional nanoparticles with ultrasound imaging and SDT. PFP is a special phase-change material that can change from a liquid state to a gaseous state when external pressure increases or temperature rises, and can stably achieve phase change ability in different nanoparticle carriers[5]. In this experiment, as the power of LIFU increased, the imaging ability of CPP nanoparticles gradually became apparent, with the best results at 4 W, consistent with the phase change results under the microscope, indicating that the liquid-gas phase change of PFP under the action of LIFU enhanced the ultrasound imaging capability of the nanoparticles[6]. In addition, different concentrations of CPP nanoparticles co-cultured with 4T1 cells for different durations showed no significant toxicity reactions or cell apoptosis, indicating good biocompatibility of the nanoparticles. The sonosensitizer Ce6, derived from natural chlorophyll, has relatively good stability and rapid clearance speed in vivo. However, the poor water solubility of Ce6 makes it unable to ensure its accumulation in the tumor area when used alone, resulting in a significant reduction in sonodynamic effect[7]. In this study, the CPP nanoparticles triggered under the same LIFU conditions produced higher levels of ROS and SDT effects than other groups, demonstrating effective tumor cell killing ability, confirming that CPP nanoparticles can maximize the effect of Ce6 through the tumor’s high permeability and retention effect, consistent with previous research results[8-9].
Abnormal proliferation of tumor tissues and their distant metastasis are fundamental characteristics of malignant tumors. The ability of cancer cells to migrate is often used as an indicator of their metastatic capability. Inhibiting the metastatic ability of breast cancer is a challenge in its treatment. The results of the cell migration experiment in this study indicate that the combined action of CPP nanoparticles and LIFU can inhibit the metastasis of breast cancer cells at the in vitro level, possibly due to: ① the killing of tumor cells by SDT also inhibits the proliferation capacity of the cells, leading to a corresponding decrease in their metastatic ability; ② the unique cavitation effect of ultrasound specifically opens cell membranes, enhancing the effect of ROS and directing it towards migration-related cell factors and pathways, thereby preventing tumor cell metastasis to some extent[10].
In conclusion, this study successfully prepared the integrated diagnostic and therapeutic Ce6@PFP/PLGA nanoparticles, confirming that under LIFU irradiation, these nanoparticles can simultaneously achieve ultrasound imaging, inhibit the growth and metastasis of breast cancer cells, providing cellular-level evidence for utilizing multifunctional nanoparticle platforms to achieve integrated tumor diagnosis and treatment. However, this study has not sufficiently evaluated the cellular uptake pathways of CPP nanoparticles, and further in-depth research on their mechanisms of inhibiting tumor cell growth and metastasis is needed; also, the in vivo ultrasound imaging, therapeutic effects, and related biocompatibility of these nanoparticles have not been confirmed, which will be further improved in subsequent experiments.