Subcellular organelles in cells often have intricate three-dimensional structures and biological functions. Observing the three-dimensional distribution and dynamics of organelles using fluorescence microscopy helps understand cellular functions and reveals interactions between organelles. However, fluorescence microscopy is limited by optical diffraction and the numerical aperture (NA) of the objective lens, resulting in blurred low-resolution images. Due to the axial resolution of wide-field and confocal microscopy (approximately 500-700 nm) being significantly lower than its lateral resolution (approximately 200-300 nm), imaging technologies that enhance axial resolution first emerged in the 1990s: In 1992, Stefan Hell developed the 4Pi microscopy technique based on point-scanning confocal microscopy, utilizing two opposing objective lenses to collect two counter-propagating wavefronts and interfere them, compressing the point spread function (PSF) of the imaging system, thereby increasing axial resolution by 5-7 times compared to wide-field microscopy【1,2】. In 1995, Mats Gustafsson proposed the I5M imaging technique based on wide-field illumination, which, under the architecture of opposing objective lenses, formed standing wave illumination and fluorescence interference wide-field detection through partially coherent light, also increasing the axial resolution by 5-7 times compared to wide-field microscopy【3,4】.
Entering the 21st century, researchers have developed numerous super-resolution imaging technologies that break the optical diffraction limit, including stimulated emission depletion (STED), single-molecule localization microscopy (SMLM), and structured illumination microscopy (SIM). Combining 4Pi technology with these super-resolution techniques has led to a series of super-resolution microscope systems with three-dimensional isotropic resolution. The first category is combined with STED technology: In 2008, Stefan Hell’s research group proposed isoSTED, which utilized 4Pi technology to compress the size of the three-dimensional annular depletion spot, achieving 50 nm isotropic resolution【5】; in 2016, Stefan Hell’s research group developed 4Pi-RESOLFT applicable to live cells using reversible fluorescent proteins【6】; in 2021, Joerg Bewersdorf’s research group developed AO isoSTED, achieving three-dimensional 50 nm resolution on tissue samples by introducing adaptive optics technology【7】. The second category is combined with SMLM technology, utilizing 4Pi technology to collect the self-interference patterns of fluorescent molecules and infer the axial position of single molecules based on the interference phase. This includes iPALM developed by Harald Hess’s group in 2009【8】, 4Pi-SMS developed by Alexander Egner’s group in 2011【9】, and the W-4PiSMSN developed by Joerg Bewersdorf’s group in 2016 and the multicolor 4Pi-SMS developed in 2020【10,11】. The third category combines 4Pi with 3D-SIM, introducing six-beam illumination interference and fluorescence interference. In 2008, Dr. Lin Shao from Mats Gustafsson’s group developed the incoherent structured light illumination imaging interference microscopy I5S, achieving three-dimensional 100 nm isotropic resolution on fixed cells【12】.
However, among the aforementioned super-resolution imaging systems with three-dimensional isotropic resolution, isoSTED, iPALM, and 4Pi-SMS are only applicable to fixed cells; 4Pi-RESOLFT, although usable for live cells, has a slow imaging speed and can only capture a few time points, making its practical value low; I5S has the potential for live cell imaging, but the system is complex and unstable, lacking hardware and algorithm improvements over the years, with no biological application scenarios thus far. Therefore, achieving fast super-resolution imaging with three-dimensional isotropic resolution on live cells remains a long-sought goal in the field of cell biology.
On December 23, 2024, the team of Yongdeng Zhang from Westlake University collaborated with the team of Xiaoshuai Huang from Peking University, the team of Jun Chao Fan from Chongqing University of Posts and Telecommunications, and the team of Liangyi Chen from Peking University to publish a research paper titledElucidating subcellular architecture and dynamics at isotropic 100 nm resolution with 4Pi-SIM in Nature Methods. This research proposed a novel 4Pi-SIM super-resolution microscope architecture that revealed complex and fine subcellular structures at three-dimensional isotropic 100 nm resolution in different types of cells. 4Pi-SIM achieved three-dimensional isotropic 100 nm optical resolution time-lapse imaging for the first time in live cells, with imaging duration reaching several hours (500-600 time points). Additionally, 4Pi-SIM also possesses simultaneous dual-color imaging capability, enabling the capture of rapid interaction processes between different organelles in three-dimensional space. This research will also be published in Nature Methods as a research briefing titled Isotropic 100 nm resolution live-cell imaging with 4Pi-SIM.
The team carefully designed the optical and mechanical structures of the 4Pi-SIM microscope based on their research experience with 4Pi single-molecule super-resolution microscopy【11】 and Hessian structured illumination microscopy【13】, minimizing the impact of thermal fluctuations and mechanical vibrations to ensure long-term stability of six-beam interference alignment and fluorescence interference; by introducing a focus-locking module, the system can ensure precise alignment of the two objective lenses; by designing a path length difference adjustment module, the system can quickly and accurately fine-tune the path length difference of the upper and lower interference arms to zero; in addition, the system adopts a novel I2M illumination module and improves the reconstruction algorithm to adaptively estimate and compensate for phase errors caused by mismatches in system path length differences in the original data, thereby minimizing reconstruction artifacts during long-duration imaging.
Figure 1: Schematic diagram and system design of 4Pi-SIM super-resolution microscope. a, Schematic diagram of the 4Pi-SIM system. b, Design diagram of the 4Pi-SIM system.
The aforementioned improvements enable 4Pi-SIM to capture and observe various subcellular structures in three-dimensional space with excellent clarity and detail. For example, the research team successfully demonstrated the double helix structure of the synaptonemal complex in mouse spermatocytes using 4Pi-SIM. However, due to insufficient axial resolution, 3D-SIM incorrectly merged the two chromosomal structures in the axial view(Figure 2a-c). In addition, the research team observed the cell membrane structure of fixed HeLa cells using 4Pi-SIM. The cell membrane typically forms many filamentous pseudopodia with diameters ranging from 100 to 300 nm on the surface. 4Pi-SIM can clearly distinguish the hollow structures of these filamentous pseudopodia in both lateral and axial dimensions. In contrast, 3D-SIM cannot resolve the detailed features of filamentous pseudopodia in the axial view(Figure 2d-e).
Figure 2: 4Pi-SIM for three-dimensional super-resolution imaging of fixed cells. a-c, 4Pi-SIM imaging of the synaptonemal complex in mouse spermatocytes labeled with immunofluorescence. d-e, 4Pi-SIM imaging of the cytoplasmic membrane of HeLa cells labeled with immunofluorescence.
Long-duration imaging experiments require ensuring the long-term stability of the interference cavity in 4Pi-SIM and compensating for the drift of the system path length difference during the imaging process in the reconstruction algorithm. Therefore, live cell imaging is more challenging compared to fixed cells. The research team utilized 4Pi-SIM for time-lapse imaging of HeLa cells labeled on the outer membrane of mitochondria, and the reconstructed images exhibited clear hollow structures, capturing the dynamic behavior of mitochondria(Figure 3a-b). In one area, the research team observed rod-shaped mitochondria transforming into disc-like structures(Figure 3c); in another area, tubular mitochondria were observed generating nanotubules from both ends, with diameters reducing from approximately 600 nm to 150 nm(Figure 3d).
The endoplasmic reticulum has a complex three-dimensional network structure and exhibits significant dynamic characteristics within live cells. With the ultra-high spatiotemporal resolution of 4Pi-SIM, the research team observed rapid reorganization of the endoplasmic reticulum in COS-7 cells, capturing the process of tubular endoplasmic reticulum gradually forming into sheet-like endoplasmic reticulum(Figure 3e-g).
Figure 3: 4Pi-SIM for fast three-dimensional super-resolution imaging of live cells. a-d, Dynamic imaging of mitochondria in HeLa cells using 4Pi-SIM. e-g, Dynamic imaging of endoplasmic reticulum in COS-7 cells using 4Pi-SIM.
Multicolor imaging is crucial for studying dynamic interactions between adjacent organelles in three-dimensional space. The research team used the photostable green fluorescent protein StayGold and a large Stokes shift fluorescent protein (dCyOFP2s) to label samples, achieving simultaneous dual-color fluorescence signal acquisition during live cell imaging under monochromatic excitation wavelengths. By simultaneously labeling the endoplasmic reticulum and mitochondria in live cells, the research team observed the interaction between the endoplasmic reticulum and mitochondria(Figure 4a-b), capturing fusion and fission events occurring at the contact points between the two organelles(Figure 4c-d). Additionally, the research team observed the complex interweaving of the tubular endoplasmic reticulum network and microtubules in live cells, forming dynamic interconnections across the cytoplasm(Figure 4e-f). These rapid interactions facilitated the rearrangement of the two organelles(Figure 4g-h).
Figure 4: 4Pi-SIM simultaneous dual-color imaging. a-d, Dual-color 4Pi-SIM dynamic imaging of endoplasmic reticulum (magenta) and mitochondria (green) in HeLa cells. e-h, Dual-color 4Pi-SIM dynamic imaging of endoplasmic reticulum (magenta) and microtubules (green) in COS-7 cells.
The 4Pi-SIM microscope represents a significant advancement in the field of live cell three-dimensional super-resolution imaging. Since Dr. Lin Shao from Mats Gustafsson’s group developed I5S in 2008, the improved 4Pi-SIM has achieved high-quality time-lapse imaging at three-dimensional isotropic 100 nm resolution in live cells over hundreds of time points for the first time. As Marcel Proust said: “The real voyage of discovery consists not in seeking new landscapes, but in having new eyes.” The 4Pi-SIM microscope holds significant potential for elucidating fine structures and dynamic behaviors at the nanoscale, promising to provide new observational perspectives and research ideas for the development of cell biology.
Researcher Yongdeng Zhang from Westlake University, Researcher Xiaoshuai Huang from Peking University, Associate Professor Jun Chao Fan from Chongqing University of Posts and Telecommunications, and Professor Liangyi Chen from Peking University are co-corresponding authors of this paper. PhD students Ouyang Zijing from Peking University, Wang Qian from Westlake University, and Li Xiaoyu from Peking University are co-first authors of this paper. Additionally, this work received important support and assistance from Dr. Lin Shao from Yale University, the Xu Xiaochun research group from Westlake University, and the Liu Changliang research group.
The research group of Yongdeng Zhang at Westlake University (https://www.westlake.edu.cn/faculty/yongdeng-zhang.html) is continuously recruiting postdoctoral researchers, research assistants, and doctoral students. Applicants with backgrounds in biomedical engineering, physical optics, computer science, biotechnology, biochemistry, and other related fields are welcome to apply. Candidates should possess good learning abilities, independent work capabilities, and team communication skills. Interested individuals are encouraged to submit their resumes.
The research group of Xiaoshuai Huang at Peking University (https://iacm.bjmu.edu.cn/) plans to recruit 2-3 postdoctoral researchers and welcomes applicants with backgrounds in biomedical engineering, physical optics, image processing, and related fields; they are also recruiting 1 research assistant and welcome applicants with a biology background. Interested individuals are encouraged to submit their resumes.
Resume Submission(Interested individuals should send their resumes and other materials to):
https://jinshuju.net/f/ZqXwZt or scan the QR code to submit your resume
https://www.nature.com/articles/s41592-024-02515-z
Editor: Eleven
1. Hell, S. & Stelzer, E.H.K. Properties of a 4pi Confocal Fluorescence Microscope. Journal of the Optical Society of America a-Optics Image Science and Vision 9, 2159-2166 (1992).
2. Hell, S.W., Schrader, M. & van der Voort, H.T. Far-field fluorescence microscopy with three-dimensional resolution in the 100-nm range. Journal of Microscopy 187, 1-7 (1997).
3. Wilson, T., Gustafsson, M.G.L., Agard, D.A., Sedat, J.W. & Cogswell, C.J. in Three-Dimensional Microscopy: Image Acquisition and Processing II 147-156 (1995).
4. Gustafsson, M.G., Agard, D.A. & Sedat, J.W. I5M: 3D widefield light microscopy with better than 100 nm axial resolution. Journal of Microscopy 195, 10-16 (1999).
5. Schmidt, R., Wurm, C.A., Jakobs, S., Engelhardt, J., Egner, A. & Hell, S.W. Spherical nanosized focal spot unravels the interior of cells. Nature Methods 5, 539-544 (2008).
6. Bohm, U., Hell, S.W. & Schmidt, R. 4Pi-RESOLFT nanoscopy. Nature Communications 7, 10504 (2016).
7. Hao, X., Allgeyer, E.S., Lee, D.R., Antonello, J., Watters, K., Gerdes, J.A., Schroeder, L.K., Bottanelli, F., Zhao, J., Kidd, P., Lessard, M.D., Rothman, J.E., Cooley, L., Biederer, T., Booth, M.J. & Bewersdorf, J. Three-dimensional adaptive optical nanoscopy for thick specimen imaging at sub-50-nm resolution. Nature Methods 18, 688-693 (2021).
8. Shtengel, G., Galbraith, J.A., Galbraith, C.G., Lippincott-Schwartz, J., Gillette, J.M., Manley, S., Sougrat, R., Waterman, C.M., Kanchanawong, P., Davidson, M.W., Fetter, R.D. & Hess, H.F. Interferometric fluorescent super-resolution microscopy resolves 3D cellular ultrastructure. Proceedings of the National Academy of Sciences of the United States of America 106, 3125-3130 (2009).
9. Aquino, D., Schonle, A., Geisler, C., Middendorff, C.V., Wurm, C.A., Okamura, Y., Lang, T., Hell, S.W. & Egner, A. Two-color nanoscopy of three-dimensional volumes by 4Pi detection of stochastically switched fluorophores. Nature Methods 8, 353-359 (2011).
10. Huang, F., Sirinakis, G., Allgeyer, E.S., Schroeder, L.K., Duim, W.C., Kromann, E.B., Phan, T., Rivera-Molina, F.E., Myers, J.R., Irnov, I., Lessard, M., Zhang, Y., Handel, M.A., Jacobs-Wagner, C., Lusk, C.P., Rothman, J.E., Toomre, D., Booth, M.J. & Bewersdorf, J. Ultra-High Resolution 3D Imaging of Whole Cells. Cell 166, 1028-1040 (2016).
11. Zhang, Y., Schroeder, L.K., Lessard, M.D., Kidd, P., Chung, J., Song, Y., Benedetti, L., Li, Y., Ries, J., Grimm, J.B., Lavis, L.D., De Camilli, P., Rothman, J.E., Baddeley, D. & Bewersdorf, J. Nanoscale subcellular architecture revealed by multicolor three-dimensional salvaged fluorescence imaging. Nature Methods 17, 225-231 (2020).
12. Shao, L., Isaac, B., Uzawa, S., Agard, D.A., Sedat, J.W. & Gustafsson, M.G. I5S: wide-field light microscopy with 100-nm-scale resolution in three dimensions. Biophysical Journal 94, 4971-4983 (2008).
13. Huang, X., Fan, J., Li, L., Liu, H., Wu, R., Wu, Y., Wei, L., Mao, H., Lal, A., Xi, P., Tang, L., Zhang, Y., Liu, Y., Tan, S. & Chen, L. Fast, long-term, super-resolution imaging with Hessian structured illumination microscopy. Nature Biotechnology 36, 451-459 (2018).
(Scroll up and down to read)
BioART Strategic Partners
(*Ranking is not in order)
(*Ranking is not in order)
Reprint Instructions
[Non-original article] The copyright of this article belongs to the author. Personal forwarding and sharing are welcome. Unauthorized reproduction is prohibited. The author holds all legal rights, and violators will be pursued.
Recent Live Broadcast Recommendations
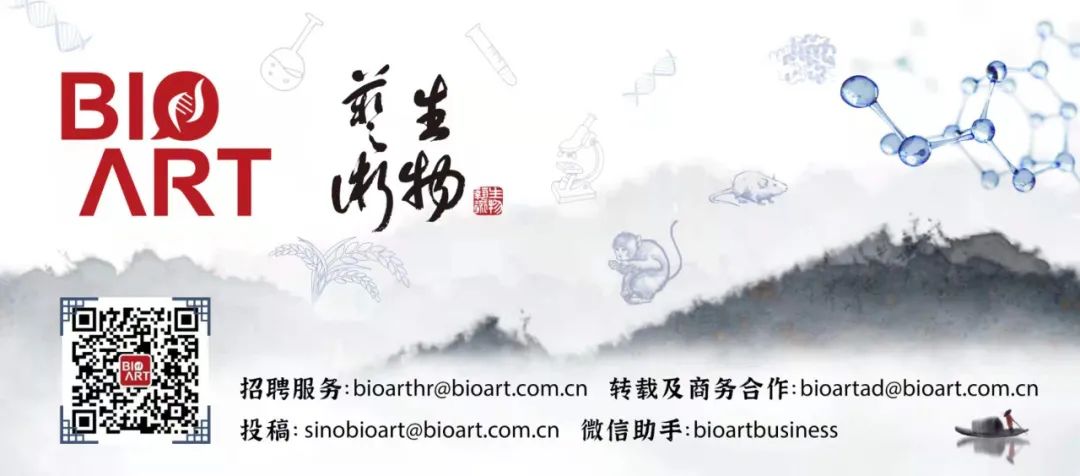