
[Source] Jiang Junde, Yang Yan, Hu Qian, et al. Research progress on the crystal transformation mechanism of phosphogypsum [J]. Ecological Industry Science and Phosphorus and Fluorine Engineering, 2024, 39(9):60-65.
[Author Introduction] Jiang Junde, engineer, engaged in the comprehensive utilization research of phosphogypsum.
[Corresponding Author] Song Fangxiang, associate professor, research direction is efficient utilization of solid waste resources.
[Funding Project] Natural Science Research Project of Guizhou Provincial Department of Education (Qian Jiao Ji [2022] No. 001)
☛(The above image is an advertisement and is not related to this article)
[Abstract] Phosphogypsum is a waste residue discharged during the wet-process phosphoric acid production and contains impurities such as soluble phosphorus, fluorine, and alkali metal salts. Simple stacking and landfill disposal of phosphogypsum will lead to problems such as cultivated land occupation and environmental pollution. The dissolution-recrystallization mechanism of phosphogypsum has always been controversial. However, converting it into different crystal forms of gypsum and applying it to different fields is currently the most promising and effective treatment method. This review summarizes the current crystallization mechanisms and controversies of converting phosphogypsum into α-hemihydrate gypsum, β-hemihydrate gypsum, and anhydrous gypsum, analyzes the commonly used preparation methods based on existing crystallization mechanisms, discusses their advantages and disadvantages, and proposes the problems and research directions to be solved in the next step.
[Keywords] phosphogypsum; crystal transformation mechanism; preparation method; research progress

Phosphogypsum mainly consists of CaSO4·2H2O, with small amounts of SiO2, P2O5, MgO, and other impurities. The wet phosphoric acid production process is the main source of phosphogypsum discharge. As one of the countries with high phosphogypsum output globally, China’s current stockpile of phosphogypsum has reached 800 million tons, and the annual generation of new phosphogypsum is about 80 million tons. Although China has abundant phosphogypsum resources, the comprehensive utilization rate is only 40%-50%. Despite numerous studies on the resource utilization of phosphogypsum, the results have not been ideal, making it difficult to fundamentally solve the comprehensive utilization problem of phosphogypsum. The resource recycling process of phosphogypsum requires certain means for its crystallization to achieve comprehensive utilization through different pathways. However, the crystallization mechanism of phosphogypsum into different crystalline forms has always been controversial, leading to the need for a large number of different crystallization agents and solvents during the crystallization process, which is poorly targeted and results in significant resource waste. This paper reviews the crystallization mechanisms and controversies of transforming phosphogypsum into α-hemihydrate gypsum, β-hemihydrate gypsum, and anhydrous gypsum, introduces the research progress of commonly used crystallization methods based on existing crystallization mechanisms, discusses their advantages and disadvantages, and proposes the next research directions to provide references for the study of phosphogypsum crystallization mechanisms and large-scale comprehensive utilization of bulk phosphogypsum.

1.1 Transformation Mechanism of α-Hemihydrate Gypsum
The mechanism of phosphogypsum transforming into α-hemihydrate gypsum is still highly controversial. The three main viewpoints of discussion are as follows: (1) Local reaction-dissolution-recrystallization mechanism; (2) Pilejter viewpoint; (3) Dissolution-recrystallization mechanism.
The first viewpoint suggests that the transformation of dihydrate gypsum to α-hemihydrate gypsum occurs in two steps. The first step is the initial stage, following local reactions; the second step is the nucleation and crystallization stage, following dissolution-recrystallization to obtain α-hemihydrate gypsum. However, BOBROV et al. proposed the difference between the transformation of single crystal dihydrate gypsum and polycrystalline dihydrate gypsum into α-hemihydrate gypsum, arguing that the transformation of single crystal dihydrate gypsum is a topological solid-state reaction process, where the c axis of the crystal remains unchanged, while polycrystalline dihydrate gypsum undergoes the three stages of gypsum dissolution, hemihydrate nucleation, and macroscopic crystal growth to complete the phase transformation into α-hemihydrate gypsum.
The Pilejter viewpoint posits that under steam pressure conditions, dihydrate gypsum first dehydrates to form anhydrous gypsum and two molecules of water; subsequently, the anhydrous gypsum combines with water molecules to form dense α-hemihydrate gypsum crystals. However, this viewpoint has not yet been experimentally proven. CHRISTENSEN et al. found that as the decomposition temperature rises, CaSO4·2H2O decomposes first into hemihydrate gypsum, followed by type III anhydrous gypsum, and finally type II anhydrous gypsum, while no diffraction peaks of anhydrous gypsum were observed before the diffraction peaks of hemihydrate gypsum appeared. Tang Mingliang analyzed through XRD experiments and found that no other phases were observed during the mutual transformation of dihydrate gypsum and hemihydrate gypsum, leading to a conclusion inconsistent with Pilejter’s viewpoint.
The third viewpoint suggests that when dihydrate gypsum is heated in saturated water vapor medium or aqueous solution, it partially dissolves in the liquid phase; another possibility is that dihydrate gypsum first loses 1.5 crystallization waters and forms hemihydrate gypsum precursors, which then dissolve in the aqueous solution. Driven by solubility differences or supersaturation, hemihydrate gypsum crystals first precipitate from the liquid phase and continuously adsorb Ca2+, SO42-, or growth units from the solution. As Ca2+ and SO42- ions continuously precipitate, dihydrate calcium sulfate will continue to dissolve and eventually recrystallize into dense α-CaSO4·0.5H2O. According to this mechanism, dihydrate gypsum undergoes a dissolution and recrystallization process during heating, forming a new crystal structure.
However, no definitive research has proven whether dihydrate gypsum first dehydrates to form hemihydrate gypsum precursors or first dissolves and then precipitates in the form of hemihydrate gypsum nuclei. Additionally, there exists a non-classical nucleation theory, which states that amorphous calcium sulfate exists before the precipitation of calcium sulfate, and the existence time of amorphous calcium sulfate is relatively short, forming calcium sulfate phases after reorganization. Therefore, the transformation mechanism of dihydrate gypsum to α-hemihydrate gypsum still requires further verification through advanced experimental analysis techniques, and the transformation process is also influenced by the types of crystallization agents, crystallization solvent systems, and impurities in gypsum. Currently, since the formation conditions of α-hemihydrate gypsum are in a saturated water vapor or aqueous solution environment, the dissolution-recrystallization mechanism is widely accepted.
In addition to the three controversial mechanisms for the formation of α-hemihydrate gypsum, other mechanisms have also been proposed. Li Ying utilized an autoclaved microcrystalline method and advanced characterization techniques to find that the transformation of dihydrate gypsum to hemihydrate gypsum involves a synergistic mechanism of in situ topological transformation and dissolution-recrystallization. In the topological reaction, the crystal axis a of dihydrate gypsum transforms into the crystal axis c of hemihydrate gypsum. According to the Hartman-Perdok theory, without external factors, hemihydrate gypsum crystals preferentially grow in a fibrous manner along the c axis. Organic crystallization agents can form network structures by complexing Ca2+ with organic acid anions, which attach to the crystal face (204), thereby delaying the growth rate of that face and reducing the length-to-width ratio of hemihydrate gypsum crystals.
1.2 Transformation Mechanism of β-Hemihydrate Gypsum
β-hemihydrate gypsum is produced by direct heating calcination in a non-saturated vapor environment. Due to its structural characteristics, β-hemihydrate gypsum powder has low strength and other disadvantages, which do not meet the quality requirements for building gypsum powder, leading to less research on the transformation of phosphogypsum to β-hemihydrate gypsum and the transformation mechanism has not been systematically studied.
Under specific conditions, phosphogypsum can transform into β-hemihydrate gypsum, involving crystallization phase transformation and hydration reactions. When phosphogypsum is exposed to certain humidity and temperature conditions, moisture is absorbed, leading to hydration reactions. During this process, the crystallization water in gypsum molecules combines with the gypsum lattice, causing the lattice structure of phosphogypsum to change from dihydrate gypsum to β-hemihydrate gypsum. This phase transformation involves changes in lattice parameters, accompanied by the contraction or expansion of the crystal. During the preparation process, grooves will form on the gypsum surface due to the release of crystallization water from within. Therefore, using a non-saturated vapor environment and direct heating calcination can yield β-hemihydrate gypsum products with specific particle morphology and groove structures. Peng Zhuofei et al. employed a raw material pre-grinding and calcination process, successfully improving the strength of gypsum by adjusting the particle size of the raw materials and the calcination temperature, meeting the strength requirements for building gypsum and showing promise for widespread application in building materials.
1.3 Transformation Mechanism of Anhydrous Gypsum
The transformation of phosphogypsum to anhydrous gypsum mainly involves calcination and liquid-phase methods. The calcination process involves dehydration, structural transformation, and phase transition processes. During heating at 100-200 ℃, phosphogypsum begins to gradually lose its crystallization water, and as the temperature rises, the dehydration process accelerates. With the removal of crystallization water, the crystal structure of calcium sulfate changes, initially forming hemihydrate calcium sulfate, and with further temperature increase, it eventually transforms into anhydrous calcium sulfate. Different heating conditions (such as temperature and time) may lead to the formation of different types of anhydrous calcium sulfate, such as α type or β type anhydrous gypsum. α type typically forms under low heating rates and high temperatures, resulting in a denser structure, while β type forms under high heating rates and low temperatures, resulting in a looser structure.
Currently, the liquid-phase method for preparing anhydrous gypsum mainly follows the dissolution-recrystallization mechanism. Chen Qianlin’s research group obtained nano anhydrous calcium sulfate in a nitric acid/sulfuric acid/alcohol solvent system. In this process, phosphogypsum is a poorly soluble electrolyte with low solubility in water, and the addition of HNO3 increases the solubility of phosphogypsum. As sulfate ions are consumed, it promotes the decomposition of calcium sulfate, leading to concentrations of Ca2+ and SO42- in the solution exceeding the supersaturation of CaSO4, resulting in the formation of CaSO4 nuclei. The crystallization of CaSO4 will also promote the further dissolution of CaSO4·2H2O, continuing in a cycle until the CaSO4·2H2O crystals are completely transformed into CaSO4 crystals. Yang Lin’s research group prepared micron-sized anhydrous calcium sulfate in a phosphoric acid/sulfuric acid solvent system, obtaining the same crystallization mechanism.
However, in the liquid-phase crystallization process in different solvent systems, the phase transition process during the dissolution of dihydrate gypsum and the loss of crystallization water has not been proven. Current conclusions cannot determine whether there exists a transformation process of dihydrate gypsum-hemihydrate gypsum-anhydrous gypsum, as dihydrate gypsum undergoes a gradual dissolution process, following the precipitation dissolution equilibrium rules, with simultaneous reverse generation processes and complex phase transition processes.

Nucleation supersaturation is the main factor affecting the competitive nucleation of different crystalline forms of calcium sulfate. According to classical nucleation theory, the smaller the molecular volume and the lower the interfacial energy, the higher the nucleation supersaturation, which is more favorable for reducing the activation energy barrier required for crystal nucleation and promoting crystal nucleation and growth. However, due to the relatively low solubility of CaSO4·2H2O crystals at room temperature, they have a relatively high nucleation supersaturation during the nucleation process, making the thermodynamically stable CaSO4·2H2O crystals easier to nucleate and grow. Non-lattice cations (all cations other than Ca2+) can effectively regulate the nucleation and growth of CaSO4·2H2O crystals and α-CaSO4·0.5H2O crystals. Introducing non-lattice cations into the crystallization system can form ion pairs with free SO42- in the solution, increasing the solubility of phosphogypsum and thus inhibiting phosphogypsum crystallization.
The thermodynamic equilibrium phase diagram of CaSO4·2H2O and α-CaSO4·0.5H2O is shown in Figure 1. It can be seen from Figure 1 that increasing the solution temperature is beneficial for the crystallization of α-hemihydrate gypsum. Adding some crystallization agents, such as salt media or alcohols (methanol, ethylene glycol, and glycerol), can effectively reduce the water activity value, facilitating the phase transition. Hydrothermal methods or atmospheric pressure salt (alcohol) solution methods essentially change the chemical environment in the reaction solution, thereby promoting the crystallization of phosphogypsum crystals. In the transformation process, without adding morphology directing agents, the resulting crystals are mostly elongated with needle-like or rod-like uniform shapes. To prepare crystals with controllable morphology and size, it is necessary to rely on crystal morphology directing agents. Morphology directing agents can be categorized into the following types: inorganic salts, organic acids and their salts, surfactants, and macromolecules. Among them, inorganic salts and organic acids (salts) are currently the most studied and have good control effects.
Figure 1 Thermodynamic equilibrium phase diagram of CaSO4·2H2O and α-CaSO4·0.5H2O
2.1 Effects of Hydrothermal Method on the Crystallization of Phosphogypsum
The hydrothermal method for crystallizing phosphogypsum involves mixing phosphogypsum with water and morphology directing agents in specific concentrations to create a slurry, which is then placed in a hydrothermal reactor to achieve controllable morphology crystal preparation. The type and concentration of morphology directing agents are important factors affecting the controllable preparation of crystal morphology. Although the hydrothermal method can produce controllable morphology and size of hemihydrate gypsum crystals, it has drawbacks such as high temperature and pressure, long preparation time, and poor operational flexibility.
Zhu Yang used phosphogypsum as raw material and prepared calcium sulfate short crystals under different inorganic chloride salt solution systems through hydrothermal methods. Yang Min et al. treated phosphogypsum with citric acid, ammonia, and lime, finding that citric acid effectively removed impurities from phosphogypsum. Chen Qianlin’s research group prepared cubic structure nano anhydrous CaSO4 under solvent thermal methods and applied it in high-density polyethylene (HDPE), resulting in an increase in elongation at break and tensile strength of the composite material by 31.43% and 9.16%, respectively, significantly impacting the properties of polymer materials.
2.2 Effects of Atmospheric Pressure Salt Solution Method on the Crystallization of Phosphogypsum
The atmospheric pressure salt solution method for crystallizing phosphogypsum involves mixing phosphogypsum with water, crystallization phase transition agents (salt media), and morphology directing agents in specific concentrations to create a slurry, which is then placed in a reaction vessel to react below the boiling point of water for a period, achieving controllable morphology crystal preparation. The main factors affecting crystal preparation during this process are reaction temperature, reaction time, type of crystallization phase transition agents (salt media), type of morphology directing agents, solution pH, and slurry ratio.
Hu Cheng et al. explored the effect of different NaCl concentrations on the crystallization water content, crystallization behavior, mineral composition, and morphology of phosphogypsum, finding that the crystallization water content of phosphogypsum gradually decreased with extended reaction time, and increased NaCl solution concentration helped accelerate the dehydration crystallization efficiency of phosphogypsum. Li Xianbo et al. studied the effects of different concentrations of sodium chloride, magnesium chloride, calcium chloride, sodium nitrate, and calcium nitrate salt solution systems on the preparation of α-hemihydrate gypsum, revealing that as the concentrations of sodium chloride, sodium nitrate, and magnesium chloride solutions increased, the rate of phosphogypsum conversion to α-hemihydrate gypsum crystals accelerated. ZÜRZ et al. converted desulfurization gypsum into α-hemihydrate gypsum in appropriate concentrations of inorganic salt solution or dilute sulfuric acid solution, finding that the product’s performance was similar to that obtained by the steam pressure method. Compared to the hydrothermal method, the atmospheric pressure salt solution method operates at lower temperatures and shorter reaction times, featuring high crystallization efficiency, uniform crystal morphology, and operational flexibility. However, introducing large amounts of salt media to reduce the water activity value in the crystallization process generally requires 5%-30% of the total mass of the slurry, and the addition of inorganic salts containing chlorine can cause severe corrosion to equipment and instruments, which is detrimental to production.
2.3 Effects of Atmospheric Pressure Alcohol Solution Method on the Crystallization of Phosphogypsum
The atmospheric pressure alcohol solution method for crystallizing phosphogypsum involves mixing phosphogypsum with water, crystallization phase transition agents (alcohols), and morphology directing agents in specific concentrations to create a slurry, which is then placed in a normal pressure reaction vessel to react below the boiling point of water for a period, achieving controllable morphology crystal preparation. The main factors affecting crystallization growth are reaction temperature, reaction time, type of crystallization phase transition agents, solution pH, and liquid-solid ratio. Excessive free hydroxyl groups in alcohol molecules can selectively adsorb on the crystal surface, inhibiting crystal nucleation and growth, thereby hindering the crystallization of hemihydrate gypsum.
Zhang Ruiheng et al. employed a non-chloride salt-alcohol solution system, using nitrate salts instead of chloride salts to reduce the amount of alcohol used, investigating the effects of liquid-solid ratio, temperature, alcohol-water ratio, zinc nitrate addition, potassium sulfate addition, and solution pH on the final product morphology, ultimately obtaining elongated columnar crystals. Compared to the hydrothermal method, the atmospheric pressure alcohol solution method overcomes the drawbacks of high temperature, high pressure, high energy density, and poor controllability, maintaining crystallization times mostly between 4-8 hours, producing uniformly shaped and sized crystals, and is environmentally friendly. However, the issue of efficient separation of alcohols introduced during the crystallization process, which generally accounts for 20%-60% of the total mass of the slurry, remains urgent to resolve due to the ease with which soluble alcohols like ethylene glycol and glycerol can form hydrogen bonds with active sites on the gypsum crystal surface, complicating conventional pressure filtration separation.
2.4 Effects of Acid Leaching Method on the Crystallization of Phosphogypsum
Li Zhan et al. used lime neutralization and acid leaching methods to remove impurities from phosphogypsum, studying the changes in the microscopic morphology of phosphogypsum during impurity treatment. The results indicated that the lime neutralization method effectively removed soluble phosphorus and fluorine impurities from phosphogypsum, but it was difficult to remove co-crystalline phosphorus; in contrast, the room temperature acid leaching method effectively removed soluble phosphorus, soluble fluorine impurities, and co-crystalline phosphorus impurities, resulting in thin-layered crystals of phosphogypsum after acid leaching. Yue Wenxiang used nitric acid leaching to prepare α-hemihydrate gypsum whiskers from phosphogypsum, finding that under the conditions of adding 33% H3PO4, 39.2% H2SO4, and stirring at 400 r/min, α-hemihydrate gypsum whiskers with a length-to-width ratio of 45 could be obtained. After surface modification with stearic acid and incorporation into polyethylene (PE), the tensile strength, flexural strength, and impact strength of the composite material were increased by 4.28%, 3.41%, and 3.98%, respectively.
3 Conclusion and Outlook
(1) The biggest obstacle to the large-scale comprehensive utilization of phosphogypsum is the unclear crystallization mechanism, leading to complex crystallization processes and high exploration costs. Currently, research on the crystallization mechanisms of phosphogypsum under different conditions is based on the dissolution-recrystallization mechanism, limited in its ability to clearly describe the generation of new phases and the transformation of original phases during the crystallization process.
(2) The lack of systematic research on the nucleation mechanism of phosphogypsum crystallization significantly affects its fine research and application. Utilizing advanced characterization techniques, such as in situ transmission electron microscopy to observe phase changes during the crystallization process, can deepen the study of the effects of different types of crystallization agents, crystallization environments, ions, and impurities on crystallization mechanisms, product morphology, and product crystal types; employing advanced techniques such as cryo-electron microscopy can provide insights into the molecular or ionic interactions during the crystallization process of phosphogypsum.
(3) The high-quality utilization of phosphogypsum requires the support of crystallization technology. Developing mild, low-cost, and simple crystallization technologies will help promote the comprehensive utilization process of phosphogypsum.
(4) Impurities can selectively adsorb on the surface of calcium sulfate crystals, altering the surface energy of the crystals and affecting the growth rates of different crystal faces, thus impacting the shape and size of the crystals. Optimizing existing production processes can reduce phosphogypsum discharge at the source or improve the quality of by-product phosphogypsum.
(5) Currently, the utilization rate of phosphogypsum remains low. Expanding the comprehensive utilization pathways of phosphogypsum can accelerate its utilization, such as in the fields of polymer materials and phase change energy storage materials.
(6) At present, the discharge of phosphogypsum exceeds its utilization, and existing comprehensive utilization technologies for phosphogypsum cannot achieve large-scale, low-cost comprehensive utilization. Therefore, developing harmless storage technologies is necessary and will be a key research direction in the future.
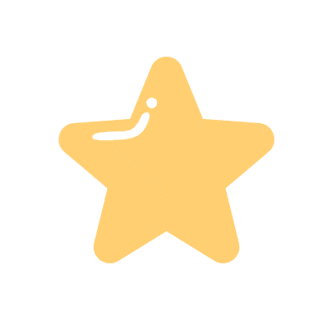

[1] Chen Yan, Yue Wenhai, Dong Ruolan. Gypsum building materials [M]. 2nd ed. Beijing: China Architecture & Building Press, 2012: 168.
[2] Yang LC, Guan BH, Wu ZB. Characterization and precipitation mechanism of α-calcium sulfate hemihydrate growing out of FGD gypsum in salt solution [J]. Science in China (Technological Sciences), 2009, 52(9): 2688-2694.
[3] Wang Zhi, Zou Aihong, Li Guozhong, et al. Recent progress in research of high strength gypsum materials [J]. New Building Materials, 1999(9): 47-48.
[4] Bobrov BS, Romashkov AV, Andreeva EP. Mechanism of Formation and Growth of alpha-hemihydrate Crystals [J]. Inorganic Materials, 1987, 23(3): 437-439.
[5] Christensen AN, Olesen M, Cerenius Y, et al. Formation and transformation of five different phases in the CaSO4·H2O system: crystal structure of the subhydrate β-CaSO4·0.5H2O and soluble anhydrite CaSO4 [J]. Chemistry of Materials, 2008, 20(6): 2124-2132.
[6] Tang Mingliang. Crystallization kinetics and preparation of α-CaSO4·0.5H2O [D]. Nanjing: Nanjing Tech University, 2010.
[7] Fu HL, Guan BH, Wu ZB. Transformation pathways from calcium sulfite to α-calcium sulfate hemihydrate in concentrated CaCl2 solutions [J]. Fuel, 2015, 150: 602-608.
[8] Mou Guodong, Ma Zesheng, Shi Nicheng. Morphological investigations of the bassinites by electronic microscope and their formation mechanism analysis [J]. Mineralogy and Petrology, 2000, 20(3): 9-13.
[9] Wang YW, Kim YY, Christenson HK, et al. A new precipitation pathway for calcium sulfate dihydrate (gypsum) via amorphous and hemihydrate intermediates [J]. Chemical Communications, 2012, 48(4): 504-506.
[10] Jia Caiyun. Crystallography of gypsum utilization—mechanism investigation on nucleation and initial growth of calcium sulfate [D]. Hangzhou: Zhejiang University, 2021.
[11] Li Ying. Study on preparation and mechanism of a high-strength gypsum from industrial by-product gypsum by autoclaved microcrystalline method [D]. Beijing: University of Science and Technology Beijing, 2023.
[12] Peng Zhuofei, Meng Xing, Liu Chenglong. Study of process parameters for producing β type semi-hydrate gypsum powder from phosphogypsum [J]. Sulphur Phosphorus & Bulk Materials Handling Related Engineering, 2019(3): 13-18.
[13] Gong S, Li XL, Song FX, et al. Preparation and application in HDPE of nano-CaSO4 from phosphogypsum [J]. ACS Sustainable Chemistry & Engineering, 2020, 8(11): 4511-4520.
[14] Zhang Guidong. Preparation of type II anhydrous gypsum by calcination of phosphogypsum and liquid phase method [D]. Guiyang: Guizhou University, 2023.
[15] Rashad MM, Mahmoud MHH, Ibrahim IA, et al. Crystallization of calcium sulfate dihydrate under simulated conditions of phosphoric acid production in the presence of aluminum and magnesium ions [J]. Journal of Crystal Growth, 2004, 267(1): 372-379.
[16] He SL, Oddo JE, Tomson MB. The nucleation kinetics of calcium sulfate dihydrate in NaCl solutions up to 6 m and 90 ℃ [J]. Journal of Colloid and Interface Science, 1994, 162(2): 297-303.
[17] Jia CY, Chen QS, Zhou X, et al. Trace NaCl and Na2EDTA mediated synthesis of α-calcium sulfate hemihydrate in glycerol-water solution [J]. Industrial & Engineering Chemistry Research, 2016, 55(34): 9189-9194.
[18] Mao JW, Jiang GM, Chen QS, et al. Influences of citric acid on the metastability of α-calcium sulfate hemihydrate in CaCl2 solution [J]. Colloids and Surfaces A: Physicochemical and Engineering Aspects, 2014, 443: 265-271.
[19] Wu XQ, Wang KM, Xiong ZY, et al. Solubility of α-calcium sulfate hemihydrate in Ca-Mg-K chloride salt solution at (353.0 to 371.0) K [J]. Journal of Chemical & Engineering Data, 2013, 58(1): 48-54.
[20] Chen YR, Zhou YL, Yang SY, et al. Novel bone substitute composed of chitosan and strontium-doped α-calcium sulfate hemihydrate: Fabrication, characterization and evaluation of biocompatibility [J]. Materials Science and Engineering C, 2016, 66: 84-91.
[21] He H, Dong FQ, He P, et al. Effect of glycerol on the preparation of phosphogypsum-based CaSO4·0.5H2O whiskers [J]. Journal of Materials Science, 2014, 49(5): 1957-1963.
[22] Zhao WP, Wu YM, Xu J, et al. Retracted article: Effect of ethylene glycol on hydrothermal formation of calcium sulfate hemihydrate whiskers with high aspect ratios [J]. RSC Advances, 2015, 5(62): 50544-50548.
[23] Sun HJ, Tan DY, Peng TJ, et al. Preparation of calcium sulfate whisker by atmospheric acidification method from flue gas desulfurization gypsum [J]. Procedia Environmental Sciences, 2016, 31: 621-626.
[24] Sun Xiangbin. Study on preparation of structure and morphology of calcium sulfate hemihydrate based on phosphogypsum calcium sulfate dihydrate [D]. Hefei: Hefei University of Technology, 2020.
[25] Yuan ZT, Wang XL, Han YX, et al. Preparation of ultrafine calcium sulfate whiskers by hydrothermal synthesis [J]. Journal of Northeastern University (Natural Science Edition), 2008, 29(4): 573-576.
[26] Fu L, Xia W, Mellgren T, et al. Preparation of high percentage α-calcium sulfate hemihydrate via a hydrothermal method [J]. Journal of Biomaterials and Nanobiotechnology, 2016, 8(1): 36-49.
[27] Wang XS, Zhou M, Ke X, et al. Synthesis of alpha hemihydrate particles with lithium and carboxylates via the hydrothermal method [J]. Powder Technology, 2017, 317: 293-300.
[28] Hrncic MK, Kravanja G, Knez Z. Hydrothermal treatment of biomass for energy and chemicals [J]. Energy, 2016, 116: 1312-1322.
[29] Shandilya M, Rai R, Singh J. Review: Hydrothermal technology for smart materials [J]. Advances in Applied Ceramics, 2016, 115(6): 354-376.
[30] Zhu Yang. Preparation of phosphogypsum-based calcium sulfate short whisker and the application research in PVC [D]. Wuhan: Wuhan University of Technology, 2018.
[31] Yang Min, Pang Ying. Investigation of cement retarder made of chemically pretreated phosphogypsum [J]. Journal of Lanzhou University of Technology, 2007, 33(6): 58-60.
[32] Li XB, Zhang Q, Hou B, et al. Flotation separation of quartz from collophane using an amine collector and its adsorption mechanisms [J]. Powder Technology, 2017, 318: 224-229.
[33] Hu Cheng, Liu Meng, Xiang Weiheng, et al. Effect of NaCl solution concentration on the transcrystallization behavior of α-hemihydrate phosphogypsum [J]. Inorganic Chemicals Industry, 2024, 56(6): 87-93.
[34] Li Xianbo, Ye Junjian, Wang Xianchen, et al. Effect of salt medium on preparation of α-hemihydrate from phosphogypsum using salt solution method [J]. Conservation and Utilization of Mineral Resources, 2017(6): 79-86.
[35] ZÜRZ A, Odler I, Thiemann F, et al. Autoclave-free formation of α-hemihydrate gypsum [J]. Journal of the American Ceramic Society, 1991, 74(5): 1117-1124.
[36] Zhang Ruiheng. Basic Study on Preparation of high-strength gypsum by phosphogypsum in alcohol-non-chloride system [D]. Kunming: Kunming University of Science and Technology, 2021.
[37] Zhang Ruiheng, Zheng Guangya, Wei Xing, et al. Research on the Production of α-Hemihydrate Gypsum from Phosphogypsum of Alcohol-Non-Chlorine Salt System [J]. Non-Metallic Mines, 2021, 44(1): 16-19.
[38] Li Zhan, Chen Jiang, Zhang Qin, et al. A Study on the removal of phosphorus and fluorine impurities from phosphogypsum [J]. Acta Mineralogica Sinica, 2020, 40(5): 639-646.
[39] Yue Wenxiang. Study on polyethylene (PE) composites modified by α-hemihydrate phosphogypsum whisker [D]. Guiyang: Guizhou University, 2017.
Research progress on crystal transformation mechanism of phosphogypsum
JIANG Junde1,YANG Yan2,HU Qian1,HE Xi1,XIA Zhichen1,XIANG Shuanglong1,SONG Fangxiang2,3(1. Guizhou Kailin Group Co.,Ltd.,Guiyang 550025,China;2. School of Chemistry and Chemical Engineering,Guizhou University,Guiyang 550025,China;3. Collaborative Innovation Center of Guizhou Province for Efficient Utilization of Phosphorus and Fluorine Resources,Guizhou University,Guiyang 550025,China)
Abstract:Phosphogypsum is a waste residue discharged during the wet-process phosphoric acid production and contains impurities such as soluble phosphorus,fluorine and alkali metal salts.Simple stacking and landfill disposal of phosphogypsum will lead to problems such as cultivated land occupation and environmental pollution.The dissolution-recrystallization mechanism of phosphogypsum has always been controversial.However,converting it into different crystal forms of gypsum and applying it to different fields is currently the most promising and effective treatment method.The current crystallization mechanisms and controversies of converting phosphogypsum into α-hemihydrate gypsum,β-hemihydrate gypsum and anhydrous gypsum are reviewed,the commonly used crystal transformation methods of phosphogypsum based on the existing mechanisms are introduced,their existing advantages and disadvantages are discussed,and finally the problems and research directions to be solved in the next step are proposed.
Key words:phosphogypsum;crystal transformation mechanism;preparation method;research progress
☛(The below image is an advertisement and is not related to this article)
This article is copyrighted by the editorial department of Ecological Industry Science and Phosphorus and Fluorine Engineering. For reprints or citations, please indicate that it is from Ecological Industry Science and Phosphorus and Fluorine Engineering.
Contact Information for the Editorial Department of Ecological Industry Science and Phosphorus and Fluorine Engineering:
Tel: 0371-6388 7309 6778 1991
E-mail: [email protected]
