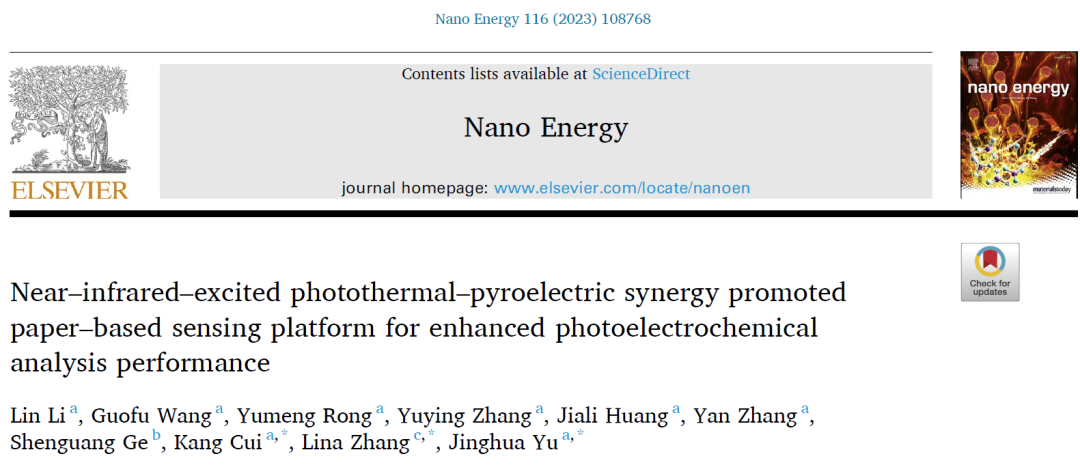
Article Information
Near-Infrared-Induced Photothermal-Pyroelectric Synergy Promotes Flexible Sensing Platform Photoelectrochemical Analysis Performance
First Author: Li Lin
Corresponding Authors: Cui Kang*, Zhang Lina*, Yu Jinghua*
Affiliation: Jinan University
Research Background
Exploring and regulating the photothermal material-driven pyroelectric effect may bring great potential for next-generation in vitro instant diagnostics. In pursuing this goal, the powerful impact of near-infrared light has largely been overlooked. Most research on photoelectric sensors has focused on visible light and ultraviolet irradiation as prerequisites for driving the conversion of light energy to electrical energy, inevitably leading to suboptimal solar energy conversion efficiency that severely hinders practical applications. The photothermal effect, caused by the unique temperature fluctuations induced by using near-infrared light to excite photothermal responsive materials as “nano-reactors,” can accelerate the migration rate of electrons at the photoelectric electrode interface. However, high temperatures typically increase the collision probability between carriers, leading to an increase in carrier recombination in the bulk of the photothermal nano-reactor. Pyroelectric materials, as a new type of material that extracts electrical energy from temperature changes, can effectively manipulate the spatial movement of electrons in their non-centrosymmetric crystal structure, generating spontaneous polarization and achieving rapid separation of temperature-induced carriers. Semiconductor materials with natural oxygen vacancies as ideal asymmetric structures for pyroelectric materials can generate positive and negative pyroelectric fields through temperature oscillations, serving as drivers for the directional migration of photogenerated carriers. However, the oxygen defects within the bandgap of pyroelectric materials can easily induce persistent photoconductive effects under short-wavelength irradiation, and sustained high-temperature fluctuations can easily damage the internal paper-based fiber structure, further leading to poor stability of the pyroelectric substrate. Therefore, exploring a simple and efficient energy-harvesting nano-system excited by near-infrared light is of great significance for eliminating adverse temperature effects and enhancing photoelectrochemical analysis performance.
Article Overview
Recently, Professor Yu Jinghua from Jinan University published an article titled “Near–Infrared–Excited Photothermal–Pyroelectric Synergy Promoted Paper–Based Sensing Platform for Enhanced Photoelectrochemical Analysis Performance” in the internationally renowned journal Nano Energy. The article constructs a flexible substrate by combining paper chips and polyimide (PI) film substrates and utilizes photothermal-pyroelectric technology to achieve energy harvesting from flexible polyimide paper electrodes. Under near-infrared radiation, the photothermally active ZnO/AgBiS2 electronic configuration and the pyroelectric performance of SrTiO3 effectors switch in real-time through fluctuations of thermal and pyroelectric fields to achieve efficient energy conversion responsive to near-infrared light.
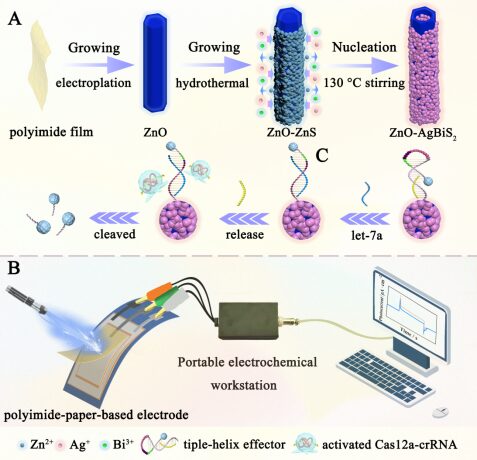
Figure 1. (A) Schematic diagram of the establishment process of the photothermal-pyroelectric sensing platform, (B) and the operation process schematic diagram.
Main Points of the Article
Point 1: Preparation and Characterization of Photothermal-Pyroelectric Interface
Using an electrodeposition process to synthesize ZnO with a large specific surface area on conductive polyimide film (PI), a sulfide layer is formed at its interface to protect ZnO from damage. Subsequently, ideal ZnO/AgBiS2 hollow nanorods are obtained on the flexible PI film through a simple ion exchange process. Scanning electron microscopy (SEM) and high-resolution transmission electron microscopy (HRTEM) show that AgBiS2 is closely supported on the ZnO nanorod skeleton through a simple ion exchange between Zn2+ and Ag+, presenting a hollow rod shape with an average diameter of about 200 nm. The pyroelectric material is synthesized under typical hydrothermal conditions in a N2 atmosphere. Notably, the microstructure and lattice of the hollow nanorods show almost no change after electrochemical conditions compared to the original ZnO/AgBiS2. Hexagonal wurtzite ZnO is observed in the XRD spectrum of the pure PI film, with an increase in sulfur content, and the apparent diffraction peak of ZnS appears successively at the interface. As the ion exchange reaction progresses, a phase transition from ZnS to AgBiS2 occurs, with the exposed (008), (110), and (118) crystal planes disappearing and AgBiS2 characteristic peaks appearing, indicating that the ion exchange reaction produces a new hybrid material.
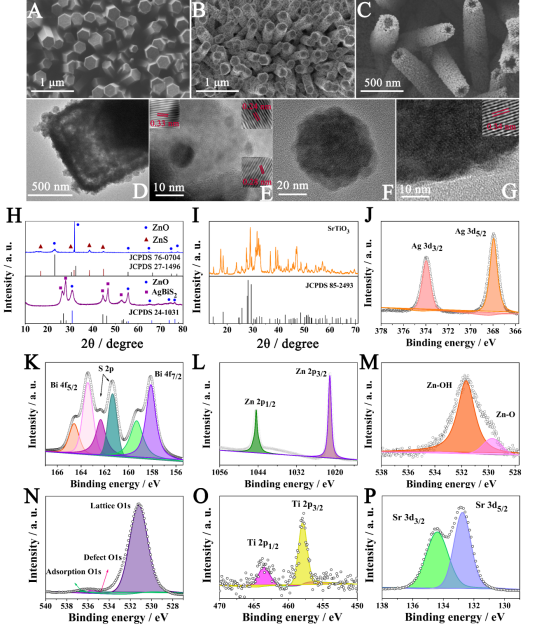
Figure 2. (A) SEM images of ZnO, (B) ZnO/ZnS, and (C) hollow ZnO/AgBiS2 nanorods. (D, E) TEM and HRTEM images of hollow ZnO/AgBiS2 nanorods and (F, G) SrTiO3 nanospheres. (H, I) XRD spectra of ZnO/ZnS, hollow ZnO/AgBiS2 nanorods, and SrTiO3 nanospheres. (J) Ag 3d, (K) Bi 4f, (L) Zn 2p, (M) Zn-O, (N) O 1s, (O) Ti 2p, and (P) Sr 3d peaks in XPS spectra.
Point 2: Light Absorption Performance of the Photothermal-Pyroelectric Sensing Platform
The pyroelectric material SrTiO3 has a significant visible light absorption edge around 400 nm. Compared to single materials, the hollow heterostructure based on AgBiS2 exhibits a wide and strong absorption peak in the near-infrared band, indicating that ZnO/AgBiS2 can output light response across the entire wavelength range, from ultraviolet to near-infrared. No significant changes are observed in the heterostructure constructed by ion exchange compared to pure samples, indicating that the internal structure is not damaged. In addition, under 808 nm laser excitation, the light response is significantly enhanced, mainly because AgBiS2 nanocrystals are typical photothermal materials with a high absorption coefficient around the 808 nm wavelength. Compared to xenon lamp irradiation, AgBiS2 can spontaneously convert light energy into thermal energy under 808 nm near-infrared irradiation without external heating facilities, which can real-time excite the pyroelectric characteristics of SrTiO3, achieving a doubling of light utilization efficiency. Moreover, the specific frequency near-infrared energy in sunlight is relatively low, and its impact on the photothermal effect at the sensing interface can be negligible.
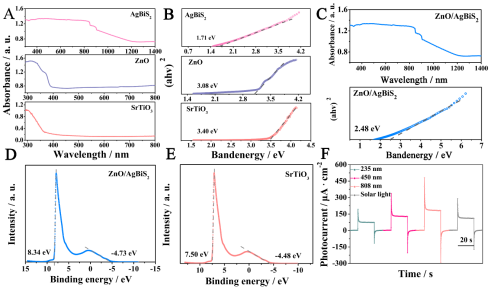
Figure 3. (A, C) DRS spectra and (B, C) Tauc plots of AgBiS2, ZnO, ZnO/AgBiS2, and SrTiO3. (D, E) UPS spectra of ZnO/AgBiS2 and SrTiO3. (F) Photocurrent response curves of ZnO/AgBiS2/SrTiO3 under different wavelength light excitation.
Point 3: Photothermal Effect of the Photothermal-Pyroelectric Sensing Platform
Under the irradiation of an 808 nm laser, the temperature of all nanocrystals shows a concentration-time-dependent temperature trend, which sharply increases and then gradually reaches equilibrium. Under xenon lamp irradiation, the temperature changes little, further proving the strong photothermal effect of near-infrared light. Interestingly, due to the surface energy of AgBiS2 nanospheres easily aggregating, leading to enhanced scattering, the photothermal effect will be significantly reduced in this case. Therefore, the thermal energy of hollow nanorods is higher than that of nanospheres, soaring by 30 °C in about 5 minutes. Based on the satisfactory thermal conductivity and heat dissipation of the PI film, the temperature returns to the initial state within 5 minutes after removing the 808 nm laser irradiation. The visualization images from the infrared thermal imager further show the temperature changes. The rapid increase in temperature proves that ZnO/AgBiS2 can quickly and effectively convert laser energy into thermal energy. Notably, this process does not exceed the Curie temperature (Tc) of SrTiO3, hence it does not damage the material, leading to failure of the pyroelectric performance due to phase change. The photothermal conversion efficiency of ZnO/AgBiS2 is 10.69%, and after multiple cycles of irradiation, it exhibits excellent photothermal stability, providing a favorable driving force for the spontaneous polarization of the pyroelectric effect.
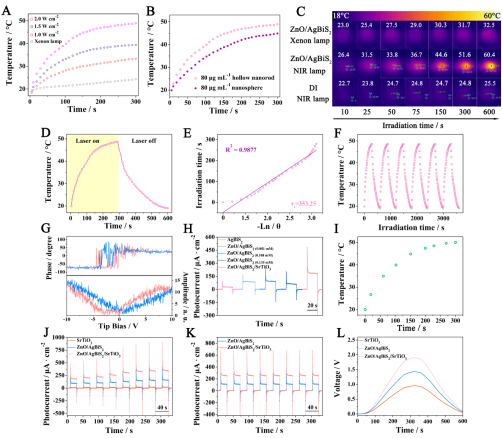
Figure 4. (A) Temperature change curves of AgBiS2 at different power densities (80μg mL−1) and (B) different morphologies. (C) Corresponding infrared thermal imager images of the solution at the end of laser irradiation (808 nm, 2 W cm−2). (D) Driving force temperature changes. (E) Linear fitting graph of –Lnθ with time during cooling. (F) Photothermal stability tests under six on/off near-infrared laser cycles. (G) Asymmetric phase curve and amplitude-hysteresis voltage curve of SrTiO3 nanospheres. (H) Changes in photocurrent response of different materials. (I) Temperature changes of the photothermal-pyroelectric platform. (J) Photocurrent responses of SrTiO3, ZnO/AgBiS2, and ZnO/AgBiS2/SrTiO3 at different temperatures and (K) constant temperature (50 °C).
Point 4: Pyroelectric Effect of the Photothermal-Pyroelectric Sensing Platform
When a voltage (10 V) is applied, the corresponding phase image and classical butterfly amplitude image clearly depict significant piezoelectricity in SrTiO3. Under the load of photothermal materials and pyroelectric materials, the photoelectric response undergoes significant changes. The increase in temperature is conducive to the separation of photogenerated electron-hole pairs and the migration of carriers to varying degrees. Notably, as the temperature changes, the current of SrTiO3 under 808 nm laser light can also reach 17 nA cm-2, although it only has a photoelectric response in the ultraviolet region, indicating that SrTiO3 can generate polarization current at continuously increasing temperatures. Interestingly, when the electrolyte temperature is kept constant at 50 °C, the current densities of ZnO/AgBiS2 and ZnO/AgBiS2/SrTiO3 are 110 μA cm-2 and 253 μA cm-2, respectively, which are both lower than the values at 50 °C constant as the temperature increases in real-time (Figure 6K). Under a heating rate of 0.03 °C s–1, the pyroelectric coefficients of SrTiO3 and ZnO/AgBiS2/SrTiO3 are 270 and 560 nC cm–2 °C–1, respectively. Temperature fluctuations are the fundamental reason for the formation of the pyroelectric field, and the pyroelectric field accelerates the migration of hot electrons, further enhancing energy release. Moreover, the pyroelectric potential of all samples shows a trend related to photothermal heating, gradually increasing with prolonged irradiation time, and ultimately recovering to the initial state under constant temperature conditions. With the loading of SrTiO3, the pyroelectric potential continuously increases, attributed to the polarization release of surface charges caused by temperature fluctuations. The process of forming interface barriers can produce energy filtering effects, significantly increasing the pyroelectric potential.
Point 5: Stability, Repeatability, and Potential Applications of the Photothermal-Pyroelectric Sensing Platform
The photocurrent curves maintain stability throughout the cyclic period, demonstrating good stability and reproducibility. When adding the same target concentration, the stimulus responses of different batches show almost no variation, and the photocurrent response value remains stable after being stored at 4 °C for 21 days. The designed photothermal-pyroelectric sensing platform can detect •OH radicals as the main reactive substance for energy conversion, which should be attributed to the dual action of the pyroelectric potential and the pyroelectric electric field. A satisfactory linear relationship is achieved between the photocurrent response and let–7a concentration in the range of 10 fM to 100 nM, with a detection limit (LOD) of 1.68 fM (S/N=3). Thanks to the special synergistic effect of photothermal and pyroelectric materials, the heterostructure drives the directional transport and separation of interlayer photogenerated hot electrons, enabling real-time modulation of the photoelectric signal in the presence of the target.
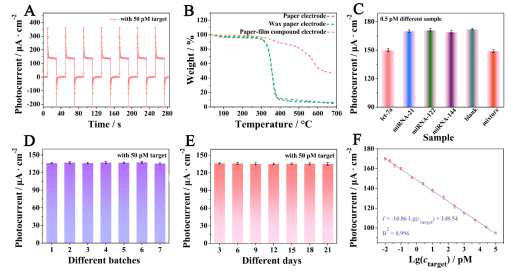
Figure 5. (A) Stability and repeatability of the photothermal-pyroelectric sensing platform and (B) flexible polyimide paper-based electrodes. (C) Selectivity study of the photothermal-pyroelectric sensing platform. (D) Photocurrent response of different batches, (E) photocurrent response after being stored at 4 °C for 21 days. (F) Calibration curve of photocurrent response for different concentrations of let-7a.
Article Link
Near–Infrared–Excited Photothermal–Pyroelectric Synergy Promoted Paper–Based Sensing Platform for Enhanced Photoelectrochemical Analysis Performance
https://doi.org/10.1016/j.nanoen.2023.108768
Corresponding Authors Introduction
Yu Jinghua Professor Introduction: Obtained a Ph.D. from the Lanzhou Institute of Chemical Physics, Chinese Academy of Sciences in 2004. Joined Jinan University in 1983. Currently a professor at the School of Chemistry and Chemical Engineering, Jinan University.
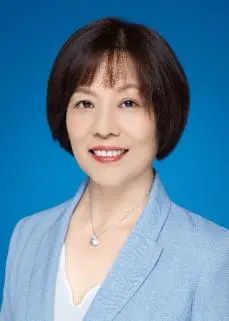
Main research directions include laboratory paper devices, functional nanomaterials, and portable electronic devices. As a corresponding author, he has published over 300 papers, with an H-index of 67.
Cui Kang Associate Professor Introduction: Cui Kang graduated from Katholieke Universiteit Leuven, Belgium, with a Ph.D. in 2014. Currently an associate professor at Jinan University.
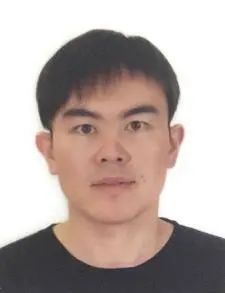
Main research directions include the synthesis of functional materials for biosensor and bioanalytical applications, as well as molecular self-assembly.
Zhang Lina Associate Professor Introduction: Zhang Lina obtained her Ph.D. in Civil Engineering from the Hong Kong University of Science and Technology in 2014. Currently an associate professor at the Shandong Provincial Key Laboratory of Building Materials Preparation and Testing, Jinan University.
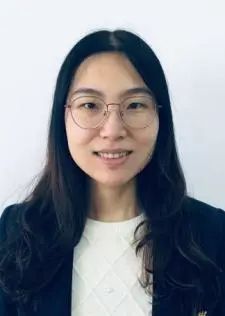
Main research directions include surface functionalization, ultra-sensitive sensor devices, and photocatalysis.
First Author Introduction
Li Lin, Jinan University, School of Chemistry and Chemical Engineering, Ph.D. student of 2020. Main research directions include portable sensing devices and applications in the photothermal-pyroelectric field. As the first author, he has published 3 SCI-indexed papers in journals such as ACS Sensors, Biosensors and Bioelectronics, and Nano Energy, and holds 2 authorized invention patents.
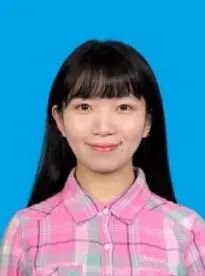
Research Group Introduction
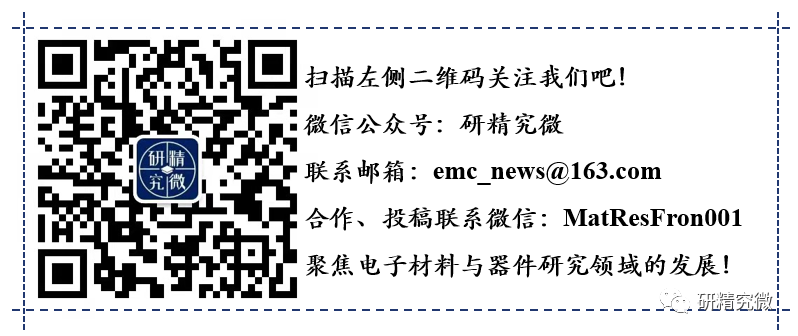