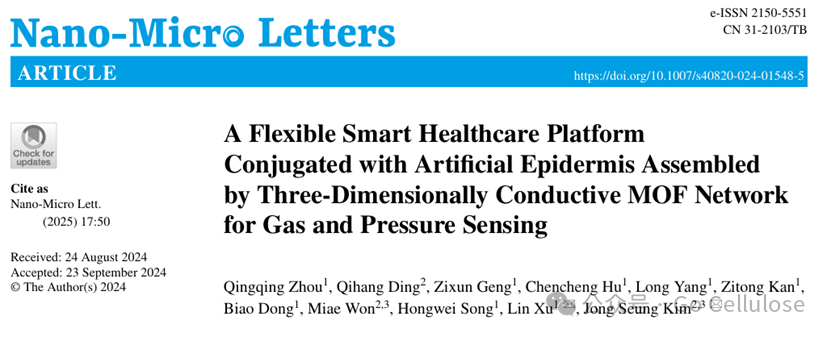
The rise of flexible, smart electronics has greatly facilitated the non-invasive and timely tracking of physiological information in remote healthcare. Carefully constructed biomimetic sensitive components are crucial for designing efficient electronic skin with advanced cognitive functions to capture diverse external stimuli. However, realistic imitation, whether in the three-dimensional interlocking hierarchical structure of the skin or in the simultaneous encoding of multi-stimulus information, remains a challenging yet essential requirement for simplifying flexible logic circuit design.
Researchers Xu Lin from Jilin University and Jong Seung Kim from Korea University constructed an artificial skin device by in-situ growing Cu₃(HHTP)₂ particles on the surface of hollow spherical Ti₃C₂Tx, simulating the stratum corneum and granule layer of the skin. The biomimetic Ti₃C₂Tx@Cu₃(HHTP)₂ exhibits independent responses to NO₂ gas and pressure, and possesses new functionalities such as acoustic feature perception and Morse code encrypted information communication. Ultimately, a wearable alarm system with a mobile application terminal was developed by integrating the dual-mode sensor into a flexible printed circuit. This system can assess risk factors associated with asthma, such as external NO₂ gas stimulation, abnormal exhalation behavior, and the force exerted by fingers, achieving a recognition accuracy of 97.6% with the assistance of machine learning algorithms.
1. Ti₃C₂Tx@Cu₃(HHTP)₂ Composite Material Characterization
SEM and TEM images demonstrate the microstructure of the prepared material, and further confirmation of the uniform distribution of elements in the composite material is provided by HRTEM and EDX characterization, proving the successful preparation of Ti₃C₂Tx@Cu₃(HHTP)₂ composite material, which resembles the epidermal structure of the skin. The tight interlocking between the granule layer (spherical Ti₃C₂Tx) and the spine layer (Cu₃(HHTP)₂ particles) can increase the contact area to effectively capture external stimuli and accelerate signal transduction. XRD was used to characterize the crystal structure of the prepared Ti₃C₂Tx@Cu₃(HHTP)₂ composite material. In the Ti₃C₂Tx@Cu₃(HHTP)₂ composite material, characteristic diffraction peaks of Ti₃C₂Tx and Cu₃(HHTP)₂ coexist, indicating that the self-assembly of conductive MOF particles does not disrupt the inherent structural integrity of the Ti₃C₂Tx framework. In summary, Cu₃(HHTP)₂ particles enter the Ti₃C₂Tx framework through electrostatic cross-linking, forming the Ti₃C₂Tx@Cu₃(HHTP)₂ composite material.
Figure 1 (a) Schematic diagram of the synthesis process of Ti₃C2Tx foam, Cu3(HHTP)2 particles, and Ti3C2Tx@Cu3(HHTP)2 composite material; (b) SEM and (c) TEM images of PMMA@Ti3C2Tx spheres; (d) SEM and (e) TEM images of hollow Ti₃C₂Tx foam; (f) SEM and (g) TEM images of Cu3(HHTP)2 particles; (h) SEM, (i) TEM, (j) HRTEM images and (k) EDX mapping images of Ti3C2Tx@Cu3(HHTP)2 composite material.
2. Biomimetic FlexibleTi₃C₂Tx@Cu₃(HHTP)₂ Sensor’s NO₂ Gas Sensing Performance
Figure 2 shows the response of the Ti₃C₂Tx@Cu₃(HHTP)₂ sensor to NO₂ gas at different concentration ranges at room temperature. The dynamic response curves of the Ti₃C₂Tx@Cu₃(HHTP)₂ sensor at different concentration ranges demonstrate its high sensitivity and fast response capability to both low concentrations (1-60ppm) and high concentrations (80-200ppm) of NO₂. Additionally, the Ti₃C₂Tx@Cu₃(HHTP)₂ sensor exhibits good gas selectivity and resistance to humidity interference. The results of repeatability and long-term stability tests show that the performance of the Ti₃C₂Tx@Cu₃(HHTP)₂ sensor remains stable after multiple uses, and the response variations at different bending angles also indicate its good bending stability. By comparing with the performance of other reported NO₂ gas sensors, this study demonstrates the superiority of the Ti₃C₂Tx@Cu₃(HHTP)₂ sensor in practical applications.
Figure 2 Dynamic response of various sensors at room temperature to low concentration range (a, 1–60ppm) and high concentration range (b, 80–200ppm) of nitrogen dioxide (NO2) gas, including Ti3C2Tx,Cu3(HHTP)2, Ti₃C2Tx@Cu3(HHTP)2 sensors; (c) Cu3(HHTP)2 and Ti₃C2Tx@Cu3(HHTP)2 sensors in relation to 1–200ppm NO2 gas; (d) Response time and selectivity of three sensors to various VOCs at 100 ppm; (f) Dynamic response test of Ti3C2Tx@Cu3(HHTP)2 sensor under various relative humidity from 11% to 85% for 100ppm NO2 gas; (g) Representative repeatability test and (h) long-term stability test of Ti3C2Tx@Cu3(HHTP)2 sensor for 100ppm NO2 gas; (i) Response of advanced chemical resistors based on NO2 to NO2 concentration and response time.
3. Ti₃C₂Tx@Cu₃(HHTP)₂ Pressure Sensor’s Piezoelectric Performance and Response Characteristics
The sensor’s good pressure sensing characteristics help distinguish weak deformation signals generated by bodily movements. Figure 3 demonstrates the piezoelectric performance and response characteristics of the Ti₃C₂Tx@Cu₃(HHTP)₂ pressure sensor. The current-voltage curve of this sensor indicates a linear decrease in resistance with increasing applied pressure, showing good ohmic contact characteristics. The Ti₃C₂Tx@Cu₃(HHTP)₂ pressure sensor benefits from the biomimetic interlocking microstructure inspired by the epidermal particle layer and the spine layer, allowing it to sensitively capture external pressure stimuli. The piezoresistive change of the Ti₃C₂Tx@Cu₃(HHTP)₂ pressure sensor gradually increases as the pressure rises from 0.6kPa to 6.1kPa, demonstrating its ability to accurately capture different pressure levels. The sensor’s response/recovery time is 0.9/0.9 s, indicating good responsiveness and recovery capability. The Ti₃C₂Tx@Cu₃(HHTP)₂ pressure sensor can withstand 300 pressure/release cycles, and throughout the sensing process, the cyclic response curve shows minimal fluctuations. Notably, with hydrophobic silicone encapsulation protection, this pressure sensor has strong capabilities to shield against moisture or environmental oxygen attacks, ensuring stable detection on easily sweating human skin.
Figure 3 (a) I-V curves at different pressures; (b) Resistance response to different pressure variables from 0.6 to 6.1kPa; (c) Response/recovery time of the Ti₃C2Tx@Cu3(HHTP)2 pressure sensor to 0.6 kPa pressure; (d) Linear curve of the Ti₃C2Tx@Cu3(HHTP)2 pressure sensor in the pressure range of 0.6~6.1 kPa; (e) Strain distribution simulation cross-section of the flexible Ti₃C2Tx@Cu3(HHTP)2 sensor under pressure; (f) Schematic diagram of the pressure sensing mechanism; (g) Repeatable response curve of the Ti₃C2Tx@Cu3(HHTP)2 sensor for 300 pressure/release cycles; (h) Dynamic pressure response of the sensor under different relative humidity (30%~90%); (i) Water contact angle test of the Ti₃C2Tx@Cu3(HHTP)2 sensor; (j) Encoding the acronym “NANO” through short and long pressure; (k) Encoding the word “MXENE” through light and heavy pressing on the Ti₃C2Tx@Cu3(HHTP)2 sensor; (l) Real-time response graph monitored by a digital multimeter, playing the classic “canon” audio.
4. Wearable and Smart Health Monitoring Application Assistant Based on Machine Learning Algorithms
By integrating the dual-mode flexible Ti₃C₂Tx@Cu₃(HHTP)₂ gas and pressure sensors into a flexible printed circuit board sized 5×2 cm², a wireless mode smart wearable alarm system was designed. By connecting to a smart terminal through a wireless WIFI module, real-time visualization of the analysis response curves of NO₂ gas and pressure can be achieved on a smartphone. By assessing external NO₂, abnormal exhalation behavior, and finger exertion levels, the smart sensing system can measure the likelihood of asthma attacks. The machine learning algorithm based on one-dimensional deep convolutional neural networks verified the accuracy of the proposed sensor in identifying breathing and bodily movement patterns, achieving an overall recognition accuracy of 97.6%. For light breathing (normal pattern), deep breathing (wheezing pattern), light and heavy pressing (limb weakness pattern), and NO₂ atmosphere, the model classification of five different breathing patterns has reached nearly 100% accuracy. The flexible smart wearable alarm system exhibits exceptional sensitivity, repeatable stability, and high precision, allowing for the analysis of detected NO₂ gas and human kinematic tremors with the aid of machine learning algorithms to perfectly distinguish similar and subtle physiological motion signals. This indicates that the designed wearable alarm system has feasible and potential application value in recording various pathological motion signals of asthma patients from multiple angles.
Figure 4 (a) Schematic diagram of the flexible smart wearable alarm system, including ESP32 chip, dual-mode sensor, power supply, and WIFI wireless data transmission; (b) Optical image showing the integration of the flexible dual-mode sensor into a flexible printed circuit to detect different pressures; (c) Real-time response of the wearable alarm system to nitrogen dioxide atmosphere, (ii, iii) normal deep breathing, (iv, v) light and heavy pressing; (d) Schematic diagram of the basic structure of the one-dimensional CNN; (e) Confusion matrix of five bodily movement patterns: S1, S2, S3, S4, and S5 represent light breathing (normal pattern), deep breathing (wheezing pattern), light and heavy pressing (limb weakness pattern), and NO2 atmosphere exacerbating asthma.
This study manufactured a biomimetic Ti₃C₂Tx@Cu₃(HHTP)₂ composite material inspired by 3D interlocking spherical structures and encoded skin information functions. This design allows for simultaneous detection of NO₂ gas and pressure, while achieving interference-free resistive output signals. Notably, the Ti₃C₂Tx@Cu₃(HHTP)₂ sensor exhibits high responsiveness, rapid response speed, 14-day long-term stability, and excellent hydrophobicity to NO₂ gas. DFT calculations further confirmed its sensing mechanism. Meanwhile, the Ti₃C₂Tx@Cu₃(HHTP)₂ sensor demonstrates effective pressure response, rapid response and recovery rates in the range of 0-6.1kPa, as well as stable repeatability over more than 300 cycles. The excellent signal transduction capability empowers flexible devices with strong functionalities in acoustic feature perception, Morse code encrypted information communication, and physiological motion perception. Finally, by combining dual-mode sensors and WIFI transmission modules, the author team developed a flexible, intelligent wearable alarm system that can accurately identify abnormalities related to asthma through machine learning algorithms. In addition to recognizing NO₂ signals and diverse bodily movements, the alarm system achieves an accuracy rate of up to 97.6% in identifying breathing and bodily movement patterns.
This paper designs a novel artificial skin device by combining conductive Cu₃(HHTP)₂ with spherical Ti₃C₂Tx, simulating the structural attributes and coding information capabilities of the skin.
This study provides a feasible strategy for developing intelligent multifunctional medical devices for emerging transformative remote medical diagnostics.
https://doi.org/10.1007/s40820-024-01548-5
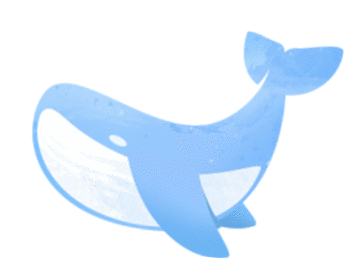
Editor: Xu Jiayue Review: Xie Rui
