The study of the provenance of metal materials is an important foundation for revealing the social structure, economic systems, and cultural exchanges of ancient societies. The large-scale, long-distance transportation and trade networks of complex societies often result in frequent mixing and remelting of metal materials during circulation. This process can destroy the original geochemical correspondence between raw mineral materials and metal products, severely hindering research on the provenance of metal materials. The Metallurgical and Materials History Research Team at Beijing University of Science and Technology has been committed to conducting data-driven materials archaeology research using machine learning algorithm models in recent years. To address the issue of metal material mixing, this research team collaborated with Peking University and the University of Illinois Urbana-Champaign to develop a methodology that combines manifold learning dimensionality reduction and Bayesian mixture models to quantitatively reconstruct the original material mixing ratios of ancient mixed metal products, successfully applying this method to research on the provenance and circulation of metal materials in Zhou Dynasty bronze artifacts. The results of this research have been published in the internationally renowned archaeological journal, Journal of Archaeological Science.
1. The Complex Mixing Problem in Ancient Metal Material Provenance Research
The development and utilization of metal resources have been important catalysts for the development of ancient civilization. During the Bronze Age, ancient people were already able to acquire metal resources over long distances through various mechanisms and exchange and redistribute them over wide areas. Therefore, the study of the provenance of metal artifacts has become an important means to reconstruct cultural interactions and socio-economic structures between ancient regions. Lead isotope analysis is the most widely used method in the provenance research of ancient metal materials. The lead isotope compositions of metal ore sources from different regions often show significant differences, and this characteristic does not undergo significant variation during human processing, providing an effective indicator for establishing the connection between mineral sources and metal artifacts. However, many civilizations during the Bronze Age had large-scale, long-distance networks for acquiring and distributing metal resources, leading to frequent mixing of metal materials from different sources during circulation, which alters their lead isotope characteristics. The ‘mixing problem’ obscures the original geochemical information of lead isotopes in metal materials, making it impossible to directly correspond with geological mineral data, severely limiting the application scenarios of lead isotope analysis in provenance research.
Previous academic approaches to the mixing problem involved searching for the ‘mixing line’ in the scatter plots of lead isotope ratios and chemical compositions to infer the characteristics of the original mixing end members. However, this method struggles to handle complex multi-endmember mixing problems and ignores the inherent dispersion and uncertainty of endmember data, limiting its ability to address practical mixing issues. In the fields of environmental science and ecological science, scholars have begun to establish Bayesian mixture models with broader applicability and stronger robustness to address similar problems, such as solving the dietary characteristics of consumers in ecosystems and the sources of environmental pollutants. This method has also been introduced into archaeology to address issues related to human and livestock livelihood patterns, population migrations, and trade networks. However, its application in the provenance research of ancient metal materials remains quite limited. The Bayesian mixture model requires determining all possible characteristics of end members that may participate in mixing as prior information. The boundaries of a small-scale mixing system are relatively easy to determine, and all potential end members within a certain geographical range can generally be input into the model. However, the long-distance metal circulation networks make it challenging to determine the boundaries of ancient metal mixing systems, as a large number of metal materials from different origins may participate as end members, posing significant challenges for model construction. This study proposes a solution to this problem: first, establish prior source groups based on manifold learning dimensionality reduction, and then further utilize the MixSIAR algorithm to establish the mixing model.
2. Model Construction
The Bayesian mixture model MixSIAR quantitatively restores the contribution of each end member to the mixed sample by using prior information such as the number of end members, analytical errors, and distribution characteristics, through Markov Chain Monte Carlo methods (MCMC Chains) for iteration, producing results in the form of probability density distribution curves, and determining whether the model has converged using the Gelman-Rubin and Geweke tests. To establish a material source end member group with sufficient coverage, this study chose to include 316 copper, tin, and lead deposits from 17 provinces that may have developed during the pre-Qin Bronze civilization in the Central Plains. Afterward, deposits with similar isotope characteristics were merged to establish the source group. The study first used geological plate or geographical boundary as grouping criteria, and the results showed significant overlaps in the lead isotope data distributions between groups, making it impossible to directly input them as end members into the MixSIAR model. Therefore, this study attempted to group them through data dimensionality reduction and clustering, identifying the inherent structure of the lead isotope data itself. The study utilized Uniform Manifold Approximation and Projection (UMAP), a novel dimensionality reduction method, to reduce the isotopic data of all deposits to two dimensions, followed by hierarchical clustering (HCA) for grouping. Two significance difference tests (ANOSIM and PERMANOVA) were used to examine the significance of differences between the preliminary grouping results, merging groups with no significant differences, ultimately defining five prior source groups (A-E) as mixed end member inputs for the model (Figure 1).
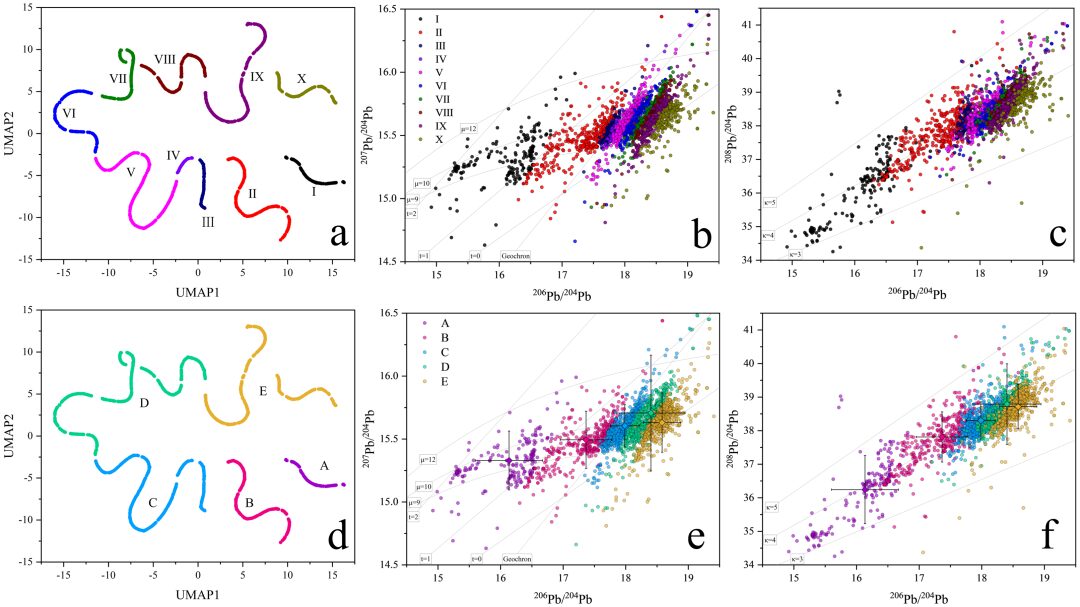
Figure 1 a: UMAP dimensionality reduction results, b-c: scatter plots of lead isotopes after HCA grouping, d: merged grouping results after significance difference tests, e-f: scatter plots of lead isotopes after merged grouping.
Using the Stacey-Kramers two-stage model method in geochemistry, the model ages (T), U/Pb (μ), and Th/Pb (κ) of the five end member groups were calculated, showing significant differences in their T values (Figure 2), indicating that the metallogenic ages of the ores correspond to the grouping results, giving them geological significance. Notably, although deposits within the same end member group may come from different geographical regions, they theoretically cannot be distinguished through lead isotope methods. Therefore, the lead isotope provenance results of metal artifacts can only be specific to particular source groups, requiring further narrowing of potential source ranges by combining other geochemical indicators and archaeological information.
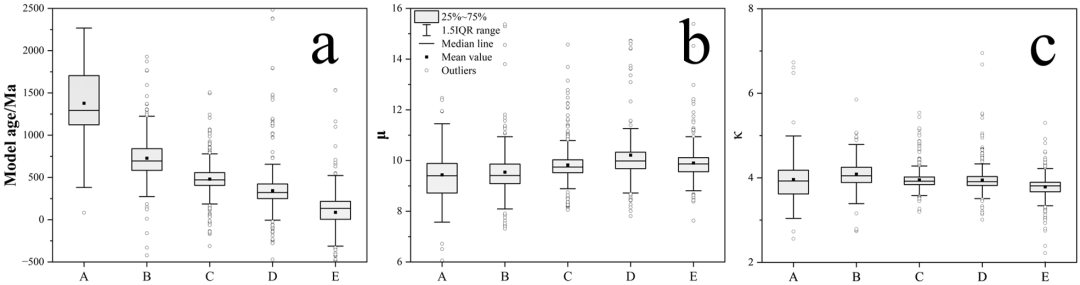
Figure 2 Distribution characteristics of T values, μ values, and κ values for each group.
The geographical distribution of each source group also exhibits certain characteristics, mainly related to the differences in tectonic ages of different regional plates in China. Figure 3 shows the proportion of each end member group in each geographical unit, with each geographical unit dominated by 1-2 end member groups, where the North China region is primarily represented by end members A and B, the Central China region (including the Qinling and Yangtze River basin) is mainly represented by end members B and C, and the South China region (including Yunnan, Guizhou, and the Nanling region) is primarily represented by end members D and E (Figure 3). Therefore, applying this model can effectively reconstruct the contribution of different geographical units to the material of bronze artifacts.
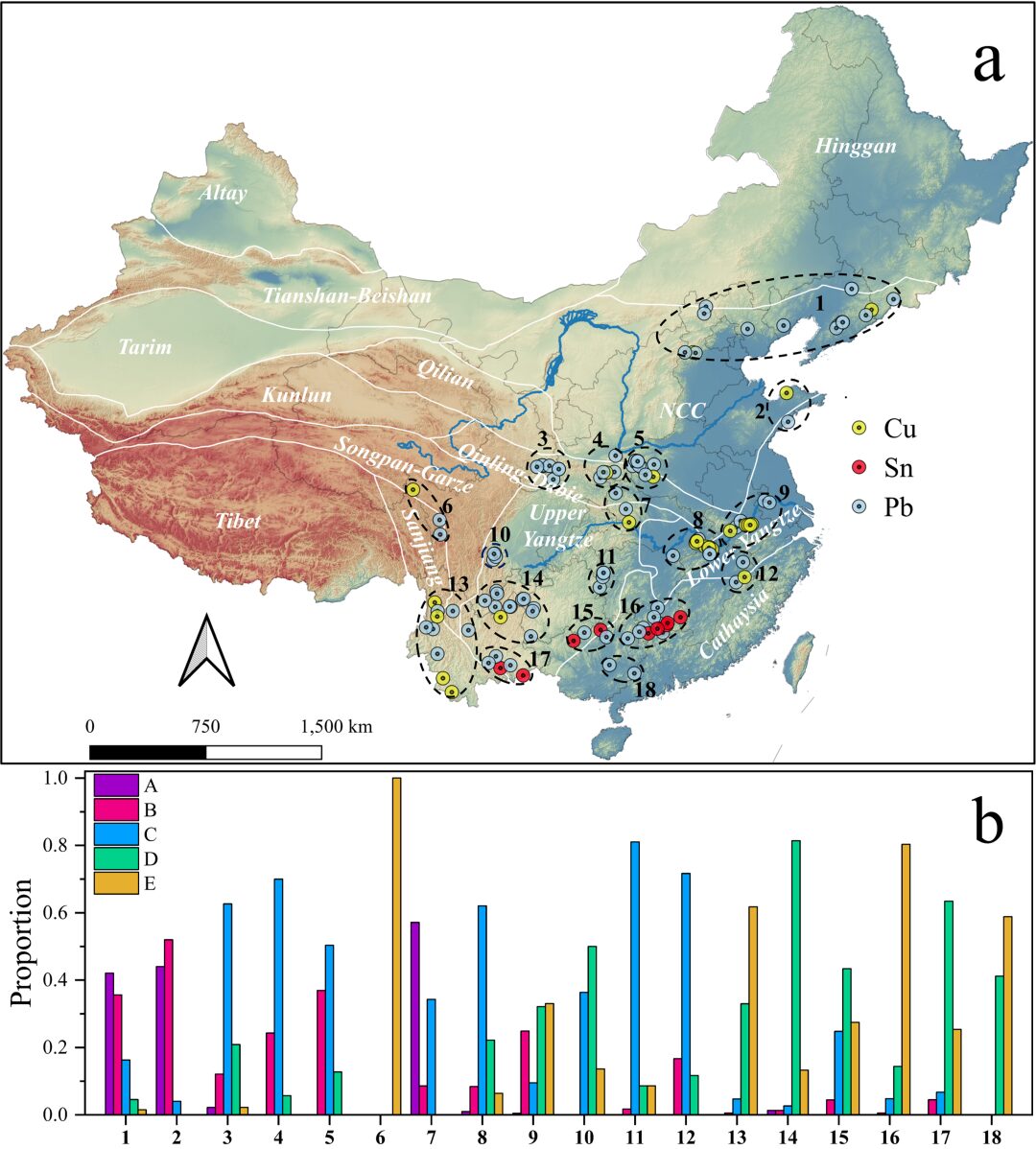
Figure 3 a: Distribution characteristics of metal deposits (copper, tin, lead) in China, b: Proportion of the five end member groups in each geographical unit.
3. The Provenance and Circulation of Metal Materials in the Zhou Period
The Zhou period had a complex political landscape and regional relationships, and as a material carrier of political power legitimacy, bronze artifacts were produced, circulated, distributed, and recast in various forms such as spoils of war, gifts, and trade goods across wide areas. Studying their material provenance has become an important pathway to understanding the political management modes and interregional relationships during the Zhou period. In this study, the Zhou period is divided into seven time periods for examination: early Western Zhou, mid-late Western Zhou, early Spring and Autumn, mid-Spring and Autumn, late Spring and Autumn, early Warring States, and mid-late Warring States.
Previous studies on lead isotope analyses of numerous Zhou bronze artifacts show that the early Western Zhou inherited the metal supply network of the late Shang Dynasty, and from the mid-Western Zhou onwards, the metal supply network gradually diversified, beginning to acquire a wider range of metal resources. Many scholars believe that the mineral resources in the middle and lower reaches of the Yangtze River, the Central China Qinling region, and the South China Nanling region played a dominant role in the metal supply network of the Zhou period, showing clear diachronic differences, revealing the changing process of the metal supply centers throughout the Zhou period, providing a good case for examining the applicability of the MixSIAR model.
Figure 4 shows that the lead isotope data of bronze artifact groups from different stages of the Zhou period exhibit continuous distribution, making it difficult to observe clear grouping, displaying significant characteristics of mixed materials. The results of the MixSIAR model calculations show that the contribution probabilities of each end member group in each period exhibit unimodal normal distributions, thus the mean and standard deviation of the probability density distribution can be used to estimate the contribution of each end member group to the bronze artifact groups in each period. The model calculation results indicate that most periods of the Zhou Dynasty had two or more major material mixing end members, confirming the high degree of mixing of metal materials during the Zhou Dynasty (Figure 5). In provenance studies, it is necessary to first demix the data and then examine its original material sources.
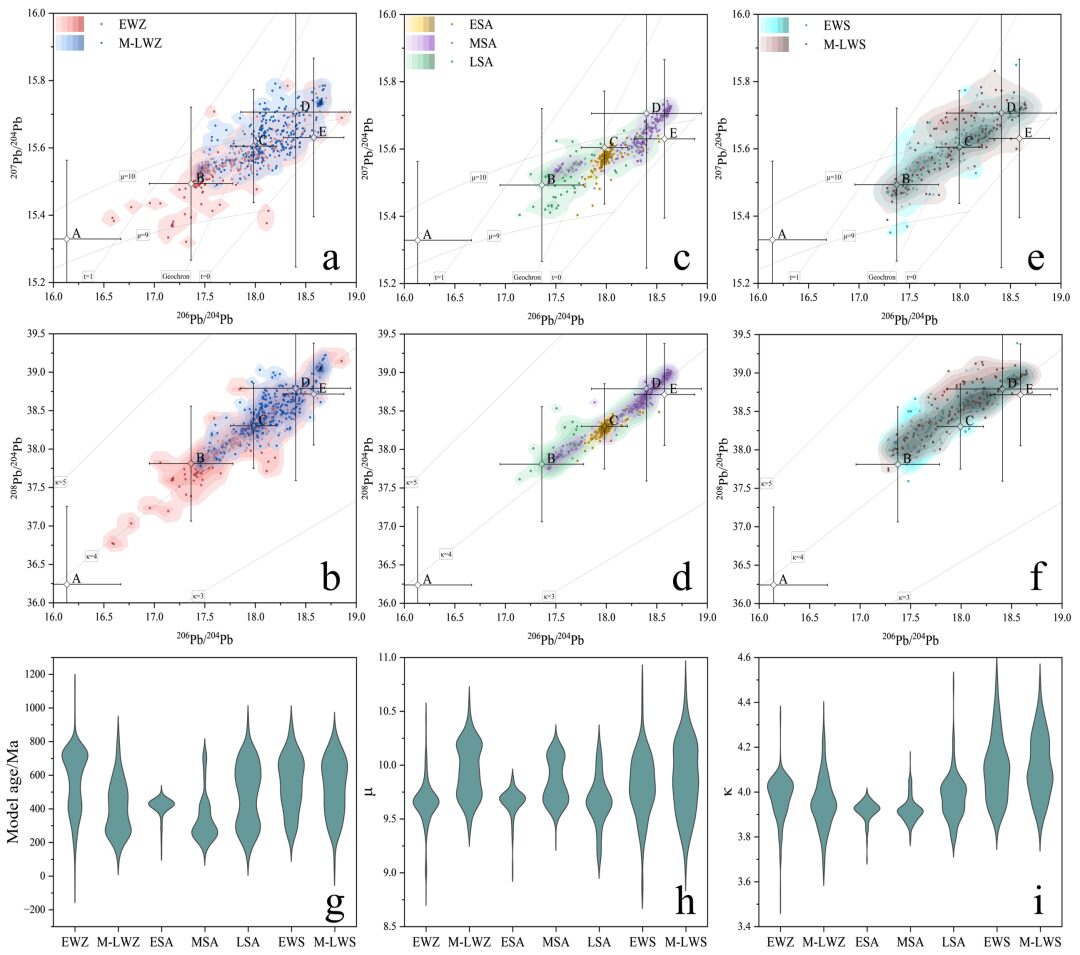
Figure 4 a-f: Distribution characteristics of lead isotopes in bronze artifact groups from various periods of the Zhou period, g-i: Distribution characteristics of T values, μ values, and κ values for each period.
End member A occupied a large proportion in the early Western Zhou and disappeared in the mid-late Western Zhou (Figure 5). This end member indicates that the ore should have been inherited from the late Shang Dynasty and was exhausted in the early Western Zhou. Previous studies have not reached a clear conclusion on the origin of this ore, while the MixSIAR model calculation results suggest that Northern North China, the Shandong Peninsula, or Northern Hubei may be its source (Figure 4).
End members B and C occupied considerable proportions throughout the Zhou period, with end member C having a greater proportion before the mid-Spring and Autumn period, while end member B had a larger proportion in the late Spring and Autumn period and thereafter (Figure 5). End member C contains multiple deposits from different geographical units, making it difficult to directly determine its origin (Figure 4). Archaeological field investigations indicate that there are numerous sites for copper mining and smelting in the middle reaches of the Yangtze River during the Western Zhou and Spring and Autumn periods, and this area is considered an important source of copper metal for the Zhou Dynasty. Although the lead content of copper deposits in the Yangtze River basin is generally low, making it difficult to affect the lead isotope results of bronze artifacts, the mining and smelting of copper may have stimulated the development and utilization of local lead resources. Early lead smelting sites dating back to the Spring and Autumn period have been discovered in the southeastern part of Hubei in the middle reaches of the Yangtze River. Therefore, it can be preliminarily judged that during the period when end member C dominated, the metal resources of the middle reaches of the Yangtze River were extensively developed and utilized and input into the Central Plains (the source represented by end member C in the early Western Zhou remains questionable). End member B includes deposits from North China, western Henan, and the lower reaches of the Yangtze River (Figure 4). Previous research results show that the Xiaoqinling area in western Henan was an important source of lead metal used from the Warring States to the Western Han period, possibly the main metal production area during the dominant phase of end member B. The diachronic change from end member C to B indicates a significant change in the metal mining and supply centers during the late Spring and Autumn period.
End member E accounted for more than 20% of the bronze artifact groups in each period, indicating that the metal resources represented by it were continuously utilized throughout the Zhou period (Figure 5). The geographical units corresponding to end member E are concentrated in southern China, including the Nanling region, Yunnan, western Sichuan, and the lower reaches of the Yangtze River (Figure 4). Numerous sites for copper mining and smelting during the Zhou Dynasty have been discovered in the lower reaches of the Yangtze River, but the lead isotope μ and κ values of its ores are generally lower than those of Zhou bronze artifacts (Figure 6). The Nanling region has a rich variety of mineral types, and several scholars have pointed out that the polymetallic resources of lead and tin in southern Hunan may have been utilized and widely disseminated to other regions of China, possibly being the main source of metal resources represented by end member E. Additionally, the lead isotope characteristics of lead-tin artifacts/pure tin artifacts from the Zhou period are also consistent with those of the Nanling metal deposits within end member E (Figure 7).
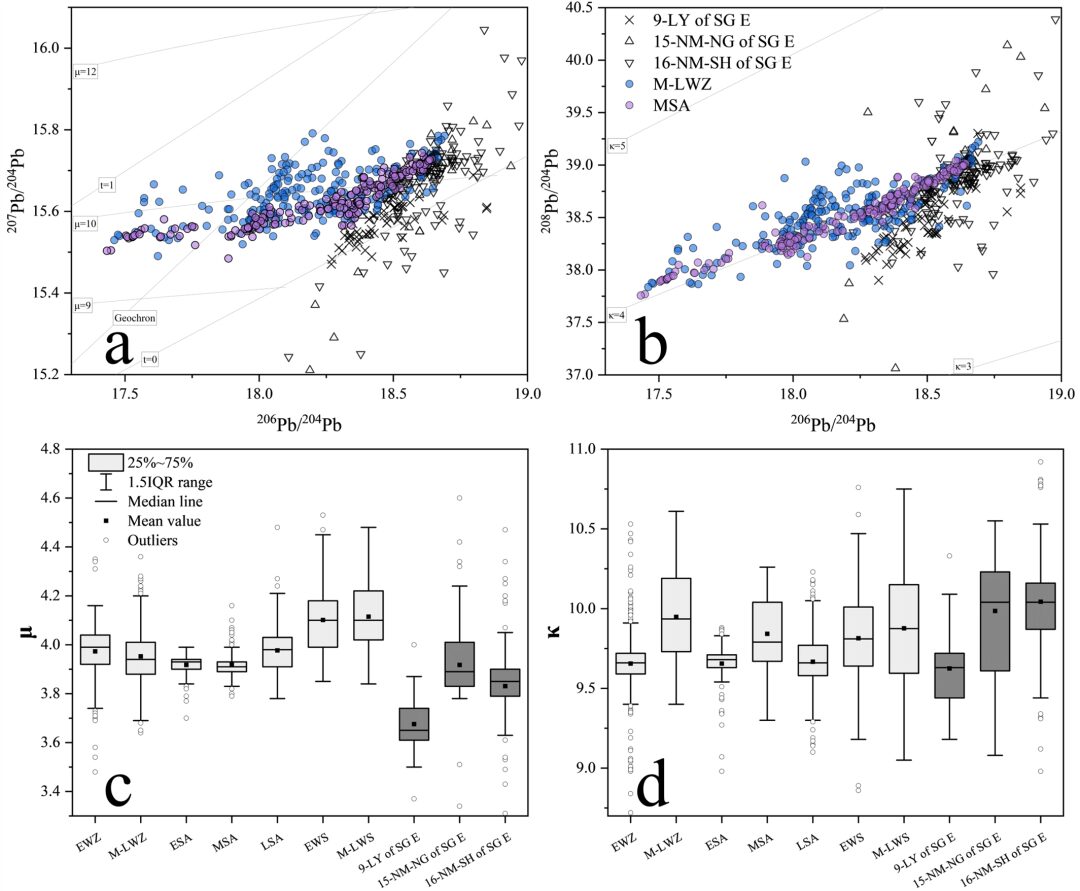
Figure 5 Proportions of each end member in the bronze artifact groups from different periods of the Zhou period.
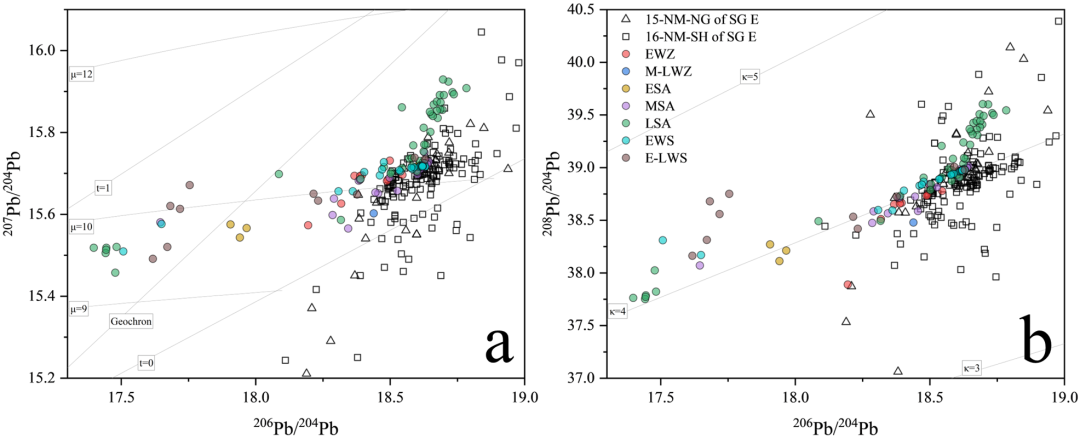
Figure 6 Comparison of lead isotopes in ores from the Nanling region and lower reaches of the Yangtze River within end member E with those of Zhou bronze artifact groups.
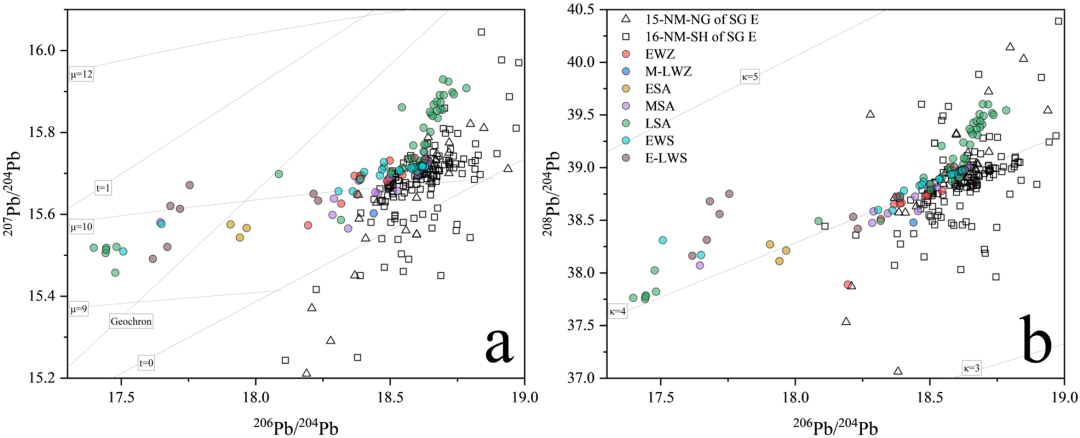
Figure 7 Comparison of lead isotopes in ores from the Nanling region within end member E with those of Zhou lead-tin artifacts/pure tin artifacts.
4. Conclusion
This study developed a novel data demixing method for lead isotopes in bronze artifacts. By employing UMAP dimensionality reduction and HCA clustering analysis, the major metal deposits in China were divided into five end members. The grouping results correspond well with the ore model ages and are closely related to the geographical units of the deposits. Based on this, the Bayesian mixture model MixSIAR reconstructed the contributions of each end member group to the bronze artifact groups at different stages of the Zhou period, revealing the complex history of metal resource development and mixing. This method also holds great potential for addressing the complex mixing and circulation issues of ancient metal materials in other regions of the world. The model output results can serve as a data foundation for further quantitative research, aiming to reconstruct and simulate the mechanisms of cultural exchanges carried by metal raw materials and artifacts among different communities over wide areas.
Article Link:
https://authors.elsevier.com/c/1gSeY15SlTyzS3
Author Biography:
Sun Zhenfei, Beijing University of Science and Technology, Institute of Science and Technology History and Cultural Heritage, PhD candidate.
Liu Siran, Beijing University of Science and Technology, Institute of Science and Technology History and Cultural Heritage, Associate Professor.
Zhang Ji, Beijing University of Science and Technology, Institute of Science and Technology History and Cultural Heritage, Associate Professor.
Chen Kunlong, Beijing University of Science and Technology, Institute of Science and Technology History and Cultural Heritage, Professor.
Brett Kaufman, Beijing University of Science and Technology, Institute of Science and Technology History and Cultural Heritage, Visiting Researcher; Assistant Professor at the University of Illinois Urbana-Champaign.
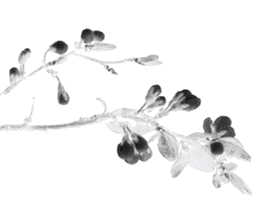
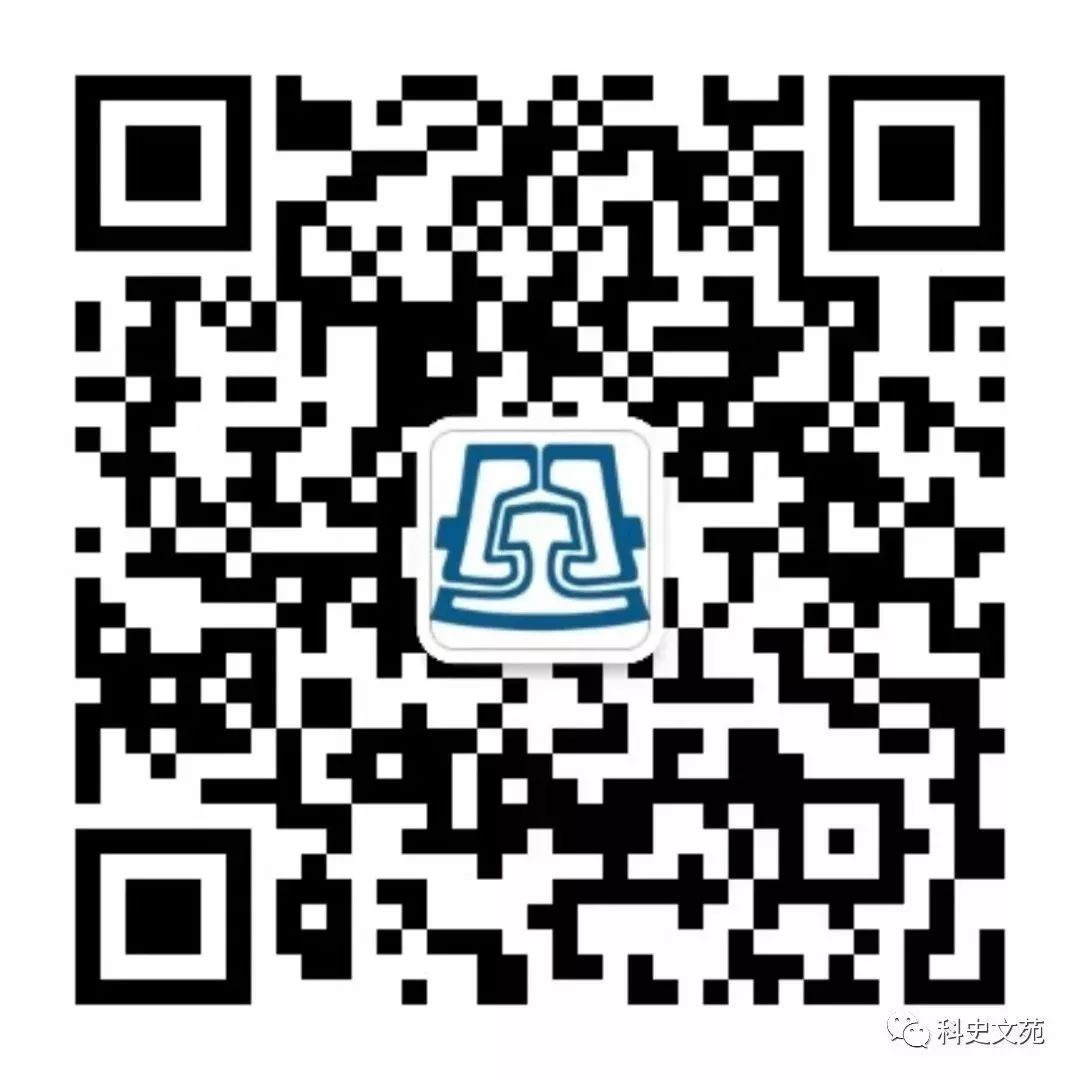
Long press the QR code to follow us