Recycling of human teeth for piezoelectric energy harvesting
Yuanyuan Yin, Shuaijie Liu, Yuehui Wang, Sihan Yang, Min Ding, Xiaohui Xu, Wei Ji, Jinlin Song
Giant 2024, 20, 100333
https://doi.org/10.1016/j.giant.2024.100333
Abstract
In the human body, biomolecules or tissues with non-centrosymmetric structures can exhibit piezoelectricity; however, there is limited research on utilizing natural tissues to manufacture piezoelectric devices for energy harvesting. Professor Jinlin Song from the Affiliated Stomatological Hospital of Chongqing Medical University and Professor Wei Ji’s team from the College of Bioengineering at Chongqing University collaborated to recycle discarded teeth and create piezoelectric nanogenerators, exploring their applications in energy harvesting. This nanogenerator can produce a stable output voltage of approximately 0.9 V under an external mechanical force of 60 N, capable of illuminating an LED light. This study employs a strategy to recycle human teeth for energy harvesting, providing a new approach to convert biological waste into green energy.
Background
Under external mechanical force, piezoelectric materials generate charges due to the deformation of internal dipoles, thus converting mechanical energy into electrical energy. Natural biological tissues or molecular materials with non-centrosymmetric structures in the human body can exhibit piezoelectricity, including bone, skin, tendons, collagen, peptides, and amino acids. The hard tissue of human teeth is mainly composed of enamel and dentin, both of which have been confirmed to possess piezoelectric properties. Many teeth are extracted in dental clinics for various reasons such as periodontitis, supernumerary teeth, impacted teeth, and orthodontic treatment, and these teeth are often treated as biological waste. Currently, there is no research exploring the piezoelectric properties of human teeth to recycle discarded teeth into valuable energy.
Illustrative Guide
This article utilizes the piezoelectric characteristics of enamel and dentin to design and manufacture a sandwich-type piezoelectric nanogenerator based on human discarded teeth, further exploring the potential of this piezoelectric device for energy harvesting (Figure 1).
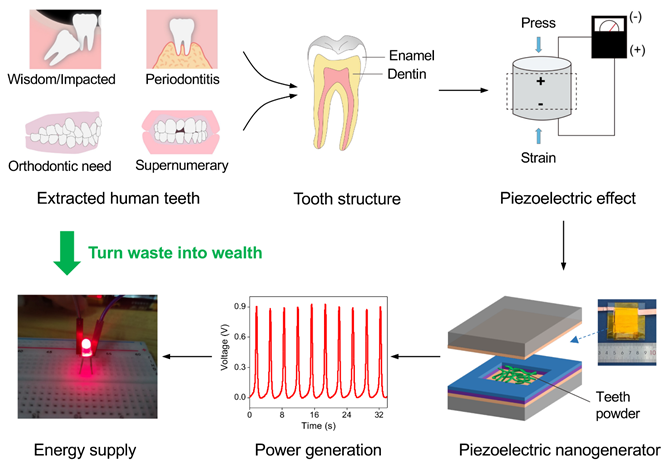
Figure 1. Schematic diagram of recycling human discarded teeth to manufacture piezoelectric nanogenerators for energy harvesting: from the piezoelectric effect of teeth, device fabrication to energy harvesting applications.
First, the quasi-static d33 method was used to study the piezoelectric responsiveness of enamel and dentin (Figure 2a). The samples were placed between the two probes of the quasi-static d33 measurement device, and the piezoelectric coefficient d33 was calculated based on the magnitude of the applied mechanical force and the amount of charge collected. Five parallel measurement experiments were conducted to determine the average d33 values for enamel and dentin, which were 1.20 and 1.64 pC/N, respectively, consistent with the range of d33 values obtained using the piezoelectric response force microscopy (PFM) testing method in the literature. The piezoelectric coefficient values of enamel and dentin are comparable to those of several other natural biological materials (Figure 2f). These results indicate that both human enamel and dentin exhibit piezoelectricity and have the potential to serve as piezoelectric biomaterials.
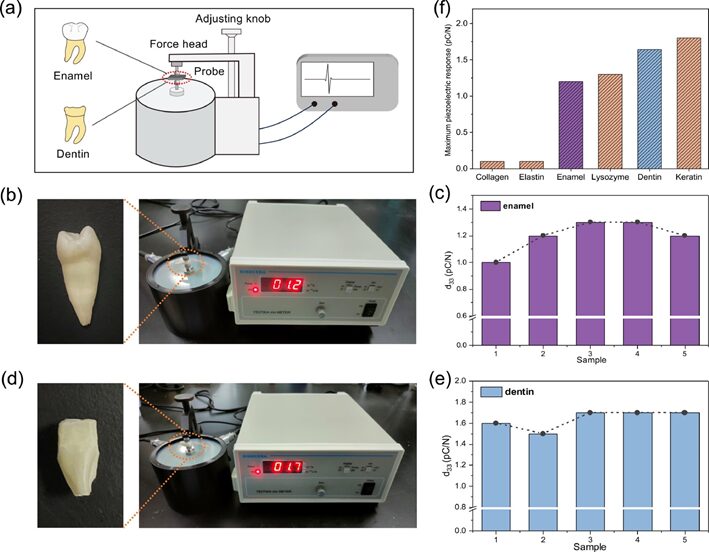
Figure 2. (a) Schematic diagram of the quasi-static d33 measurement device. (b-c) Photographs of the d33 measurement values for enamel and five parallel experimental test values. (d-e) Photographs of the d33 measurement values for dentin and five parallel experimental test values. (f) Comparison of the piezoelectricity of enamel and dentin with different biological materials.
To further investigate the manufacturing of piezoelectric devices from discarded tooth powder and assess their potential applications in energy harvesting, this study designed and prepared a sandwich-structured piezoelectric nanogenerator (Figure 3a). Figure 3b shows the schematic diagram of the power generation process of the nanogenerator under forward connection. When an external force of 10 N was applied, the piezoelectric nanogenerator generated an open-circuit voltage of approximately 0.48 V (Figure 3c). The voltage value remained constant under the same pressure cycle. When the applied pressure was increased to 20, 40, and 60 N, the generated voltages increased to approximately 0.60, 0.74, and 0.90 V, respectively (Figure 3c-g). Notably, the corresponding voltage values under different applied forces showed a good linear relationship within the range of 10 to 60 N (Figure 3h), indicating that the device exhibits stable linear piezoelectric response.
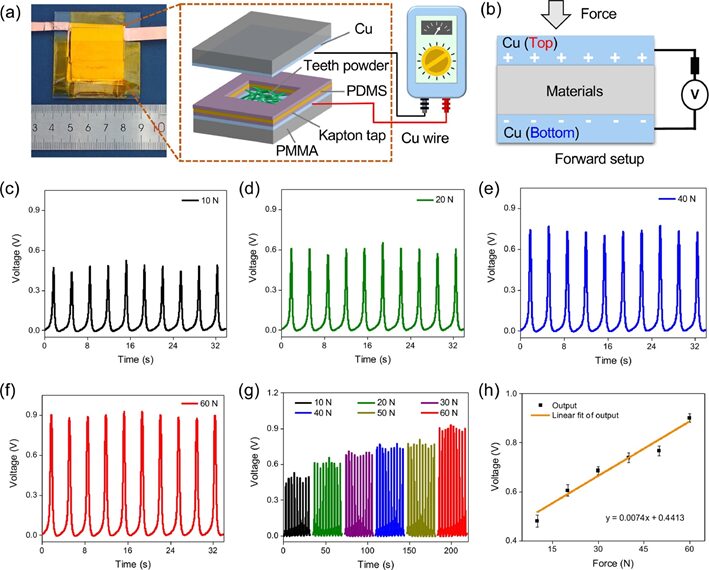
Figure 3. (a) Schematic diagram of the manufacturing process of the piezoelectric nanogenerator. (b) Schematic diagram of the piezoelectric response during forward connection. (c-f) Open-circuit voltages generated during forward connection under external forces of 10, 20, 40, and 60 N. (g) Open-circuit voltages obtained during forward connection under external forces of 10 to 60 N. (h) Linear fitting of the applied mechanical force and voltage output during forward connection.
To verify the stability of the piezoelectric nanogenerator and that the obtained electrical signals originate from the piezoelectric tooth powder, this study tested the open-circuit voltage output of the piezoelectric nanogenerator during reverse connection (Figure 4a). The voltage value obtained under a mechanical force of 10 N was approximately -0.43 V, close to the value during forward connection (Figure 4b). Similarly, under the same mechanical forces (20, 40, and 60 N), the open-circuit voltages generated by the nanogenerator during reverse connection were close to those during forward connection (Figure 4b). These results exclude the influence of contact resistance or parasitic capacitance changes. Furthermore, in reverse connection, the voltage values also exhibited a good linear relationship with mechanical force variations (Figure 4c). Under a mechanical force of 60 N, the amplitude of the output voltage signal did not show significant degradation during 1600 cycles of repeated loading tests (Figure 4d-g), indicating good durability of the device.
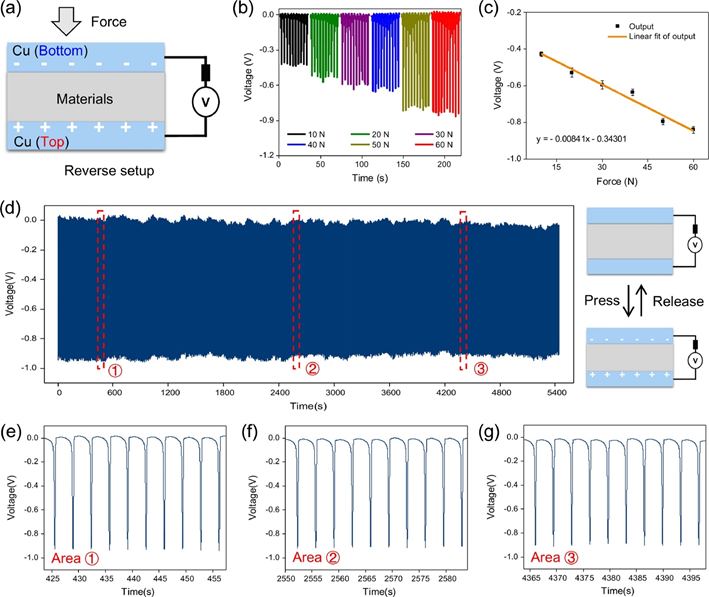
Figure 4. (a) Schematic diagram of the piezoelectric response of the nanogenerator during electrode reverse connection. (b) Open-circuit voltages obtained during reverse connection under external forces of 10 N to 60 N. (c) Linear fitting of the applied mechanical force and voltage output during reverse connection. (d) Mechanical durability test under 60 N repeated impact for 1600 times. (e-g) Piezoelectric response signals during durability tests at different time intervals.
To explore the potential applications of this piezoelectric nanogenerator in energy harvesting, this study designed and manufactured an energy harvesting system powered by an LED (Figure 5a). When force was applied to the piezoelectric nanogenerator with a finger, the power output generated by the piezoelectric device lit the LED (Figure 5b). When the finger was removed to release the pressure, the LED turned off, indicating that the electrical output came from the piezoelectric active materials in the device (Figure 5c). Pressing the piezoelectric nanogenerator again could power the LED, indicating that the device has good mechanical force response characteristics (Figure 5d). The on/off state of the LED can be controlled by different pressure-release cycles (Figure 5e), indicating the potential of this piezoelectric nanogenerator for energy harvesting applications.
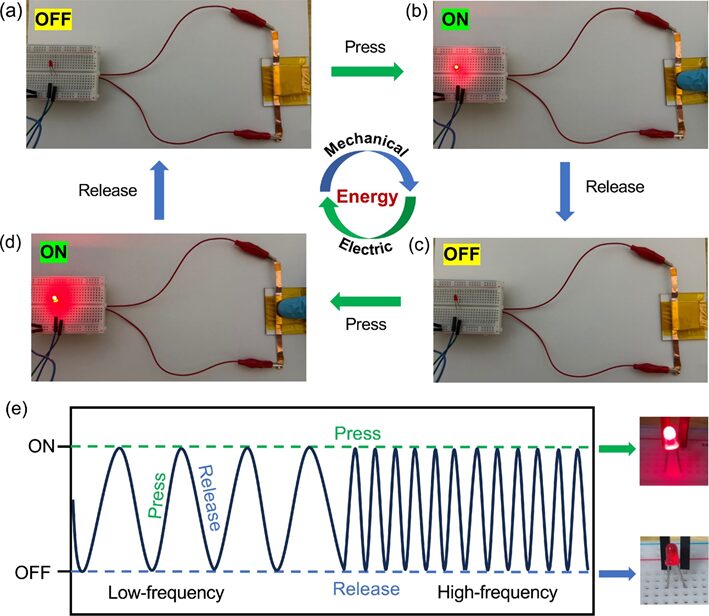
Figure 5. (a) Photo of the nanogenerator connected to an energy harvesting system containing an LED light. (b-d) Controlling the illumination of the LED light by applying and releasing pressure on the piezoelectric nanogenerator. (e) The LED light can be stably turned on and off by pressing and releasing the piezoelectric nanogenerator in different cycles.
Author Information
Yuanyuan Yin (First Author)
Postdoctoral researcher at the Affiliated Stomatological Hospital of Chongqing Medical University, focusing on the pathogenesis and treatment of periodontitis and periodontal tissue regeneration-related research.
Shuaijie Liu (First Author)
Ph.D. student of the 2023 cohort at the College of Bioengineering, Chongqing University, mainly researching the synthesis of biological piezoelectric materials and the design and application of bioelectronic devices.
Yuehui Wang (First Author)
Master’s student of the 2021 cohort at the College of Bioengineering, Chongqing University, mainly researching self-assembled biological piezoelectric materials.
Wei Ji (Corresponding Author)
Professor and doctoral supervisor at Chongqing University. His main research fields include peptide self-assembly, supramolecular hydrogels, biological piezoelectric materials, smart bionic materials, and biomedical electronic devices. He has published over 40 academic papers and hosted several national natural science foundation and Chongqing scientific research projects.
Jinlin Song (Corresponding Author)
Associate professor and chief physician at the Affiliated Stomatological Hospital of Chongqing Medical University, doctoral supervisor. His main research focuses on the pathogenesis of periodontitis and periodontal regeneration materials. He has hosted 8 national projects including NSFC (Key Class 1 project) and National Key R&D Program, and has received 7 provincial and ministerial level awards including the First Prize for Scientific and Technological Progress in Chongqing. He has published over 130 academic papers in high-level international journals as corresponding or first author.
Written by: Original authors
Edited by: Editorial Department of Giant
Original article from the Editorial Department of Giant. Personal forwarding and sharing are welcome. For reprints by publications or media, please contact via email: [email protected]
About Us
Giant is co-hosted by South China University of Technology and Elsevier, focusing on fundamental and applied polymer science, covering disciplines such as chemistry, physics, biology, and materials. The journal aims to bring a unique inclusive perspective to the development of macromolecular sciences and provide a discussion platform for new concepts and shared characteristics in macromolecular sciences. We sincerely welcome submissions.
For more information, please visit:
https://www.journals.elsevier.com/giant
Scan the QR code below to follow us QR Code
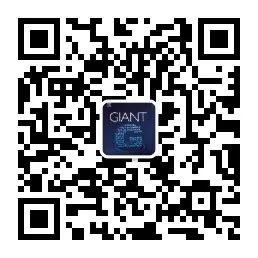