Background Introduction
Lithium-ion batteries (LIBs) have garnered significant attention regarding their performance at low temperatures, as their cycling performance declines sharply with decreasing temperature. Despite significant improvements in battery discharge capabilities in recent years, reversible charging of batteries at temperatures as low as -20°C or even lower remains challenging. The primary reason is the severe lithium plating phenomenon that occurs during charging, leading to the loss of active lithium ions and rapid capacity decay of the battery.
The enhancement of battery low-temperature performance can be achieved by optimizing the electrolyte composition. The key performance of the battery at low temperatures is closely related to the electrolyte/electrode interface, which depends on the solvent’s ability to desolvate Li+ ions during charge-discharge cycles, as well as the kinetics of ion transfer, including ion diffusion in the electrolyte and electrode materials and charge transfer at the electrode-electrolyte interface. Charge transfer is primarily influenced by the coordination environment of Li+ ions in the electrolyte. For commercially available LIBs based on graphite anodes, ethylene carbonate (EC) is an essential electrolyte component, forming a solid electrolyte interface (SEI) to ensure the battery’s ongoing stability and compatibility with other solvents. However, apart from its high melting point, EC preferentially coordinates with Li+ ions to form a tight solvation shell, resulting in a high desolvation energy barrier. In contrast, propylene carbonate (PC) has a wide liquid range (-48.8 to 242 °C) and is considered an important solvent for low-temperature electrolytes. However, PC exhibits poor electrochemical compatibility with graphite anodes, preventing its application in lithium-ion batteries.
Results Summary
The research group led by He Xiangming at Tsinghua University has made significant progress in lithium-ion battery low-temperature charging by using propylene carbonate (PC)-based electrolyte coupled with 2H/3R phase graphite. The 2H/3R phase graphite exhibits highly reversible lithium insertion/extraction behavior in both traditional EC- and PC-based electrolytes (1M LiPF6 dissolved in PC/ethyl methyl carbonate (EMC) (30:70, w/w) + 2 wt% vinyl carbonate (VC)), with Coulombic efficiencies of 94.65% and 94.98%, respectively. Soft-pack full cells (NMC622丨Gr) using the two electrolytes can stably operate for over 500 cycles, although the polarization of the battery using PC-based electrolyte is slightly lower than that using typical EC-based electrolyte (Figure 1). This result confirms the advantages of 2H/3R phase graphite as an anode, which can extend the lifespan of batteries using PC-based electrolyte. Furthermore, subsequent studies have demonstrated more advantages in low-temperature performance. At low temperatures (-20°C), the battery using PC-based electrolyte maintains a capacity retention of up to 90.2% after 30 cycles, with an average Coulombic efficiency of 99.95%. In contrast, the Coulombic efficiency of the battery using EC-based electrolyte drops sharply after the sixth cycle, with a capacity retention of only 31.20% after 25 cycles.
Conductivity tests conducted at different temperatures indicate that the ionic conductivity of both electrolytes decreases with lowering temperatures. Notably, the ionic conductivity of the PC-based electrolyte does not exceed that of the EC-based electrolyte (Figure 2c), suggesting that the ionic conductivity of the electrolyte may not be the reason for the superior low-temperature performance of batteries using PC-based electrolyte. Another significant difference between the batteries using the two electrolytes is that the discharge curve of the battery using EC-based electrolyte shows a voltage plateau at around 3.9 V (Figure 2e), indicating severe lithium plating during the previous charging process. This lithium is easily converted into inactive lithium, leading to a rapid capacity decline during cycling. After testing the battery at -20 °C and then raising the temperature to room temperature for cycling, results show that the battery using PC-based electrolyte retains 78.7% of its original capacity (a loss of 21.3%), while the battery using EC-based electrolyte retains only 39.2% of its original capacity (a loss of 60.8%) (Figures 3a and 3b). Further research using X-ray photoelectron spectroscopy to probe the SEI composition on the graphite anode (Figure 4) and molecular dynamics (MD) simulations to study the solvation structure of PC- and EC-based electrolytes (Figure 5) has been conducted. Experimental and theoretical calculations indicate that in PC-based electrolyte, more PF6– anions participate in the Li+ solvation shell, forming a favorable fluorine-rich SEI that significantly suppresses the co-insertion and decomposition of PC. Additionally, the reduction potential of Li+ ions in PC-based electrolyte is lower than that in EC-based electrolyte (Figure 6), making it less likely for lithium plating to occur during the discharge process at low temperatures.
Illustrated Guide
Figure 1. Electrochemical performance of full cells using propylene carbonate (PC) or ethylene carbonate (EC)-based electrolytes at room temperature. Normalized capacity curves of the battery at (a) the first and (b) the 500th cycle. (c) Stability and Coulombic efficiency of batteries using PC- (10PC/90EMC) or EC-based electrolyte (30EC/70EMC) during cycling at 0.5C between 2.75-4.3V.
Figure 2. Electrochemical performance of full cells using PC- or EC-based electrolytes at low temperatures. (a) Discharge capacity of the battery at different temperatures (charged at room temperature). (b) Cycling performance of the battery at -20℃. (c) Ionic conductivity of the electrolyte at different temperatures. Charge/discharge curves of batteries using (d) PC-based (20PC/80EMC) or (e) EC-based (30EC/70EMC) electrolytes.
Figure 3. Analysis of capacity loss in batteries. Capacity of full batteries using (a) PC-(20PC/80EMC) or (b) EC-based electrolyte (30EC/70EMC) after cycling tests at -20℃. Capacity loss analysis of the NCM622 positive electrode using (c) PC-(20PC/80EMC) or (d) EC-based electrolyte (30EC/70EMC) after cycling tests at -20℃. (e) Capacity loss analysis of the graphite anode after cycling tests at -20 °C. (f) Impedance measurement of the graphite anode using a button cell symmetric electrode method, where the state of charge of the graphite is 50%. All capacities are measured at room temperature and 0.1C.
Figure 4. Characterization of the graphite anode surface by XPS. (a) C 1s and (c) F 1s spectra of the graphite anode of the battery using PC-based electrolyte (20PC/80EMC). (b) C 1s and (d) F 1s spectra of the graphite anode of the battery using EC-based electrolyte (30PC/70EMC).
Figure 5. (a) MD simulation of the PC-based electrolyte (20PC/80EMC) and (b) MD simulation of the EC-based electrolyte (30EC/70EMC) (Li: green; C: gray; O: red; P: pink; F: cyan). Radial distribution function of Li+ from MD simulations: (c) with solvent molecules; (d) with PF6– anions.
Figure 6. Solvation structures of Li+ ions in (a) PC- (20PC/80EMC) and (b) EC- (30EC/70EMC) based electrolytes. ((c),(d)) Partial density of states (PDOS) of Li+ in the above two electrolytes, and (e) average energy states of solvated Li+ in the two electrolytes.
Author Introduction
Article Information
Wu Y, Zhang J, Liu J, et al. Boosting reversible charging of Li-ion batteries at low temperatures by a synergy of propylene carbonate-based electrolyte and defective graphite. Nano Research https://doi.org/10.1007/s12274-023-5968-4.
Scan the QR code or click the “Read the original text” at the bottom left to access the full text
Follow us on Bilibili, Video Number, and official website for more exciting content!
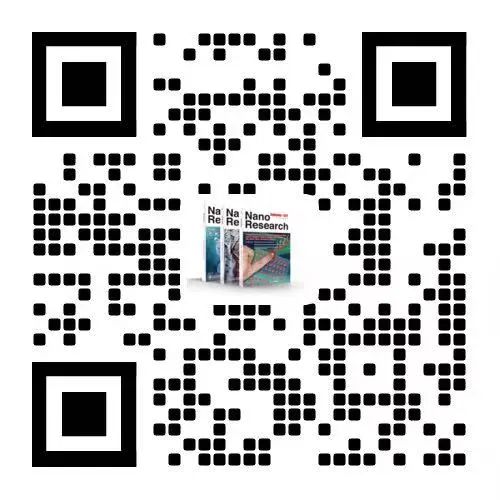
Bilibili
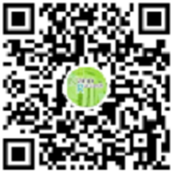
Video Number
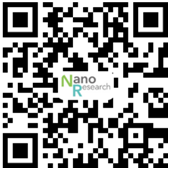
Official Website