First Author: Wang Ben (Assistant Professor, Shenzhen University), Dr. Chen Qifeng
Corresponding Author: Professor Zhang Li
Affiliation: The Chinese University of Hong Kong
The sci-fi movie “Fantastic Voyage” tells the story of five American doctors who are shrunk to a micron scale and injected into a patient’s body to perform vascular surgery, escaping through the eye after completing their mission. This film embodies humanity’s urgent desire to use micro-nano robot technology for the diagnosis and treatment of human diseases. Typically, we can classify medical robots based on their size, from large robots like the Da Vinci surgical robot and endoscopes to small micro-nano robots, each having its advantages and limitations.
Figure 1. Medical robots of different scales
For example, flexible endoscopes are common clinical tools for intraluminal surgery. They can access deep areas of the body through some natural cavities. Even so, endoscopes are still limited by their size and tethered design, restricting their ability to enter narrow and tortuous lumens.
On the other hand, micro-nano robots refer to robots that are sized at the micro-nano scale, smaller than the diameter of a hair or even a cell. Due to their small and untethered characteristics, they have the potential for high-precision movement in tortuous lumens. However, high movement precision inevitably leads to trade-offs in travel distance, making micro-nano robots very time-consuming and inefficient in long-range movements.
So, is it possible to combine the complementary advantages of endoscopes and micro-nano robots?
Figure 2. Traditional medical robots and microrobots each have their pros and cons.
Professor Zhang Li from The Chinese University of Hong Kong, along with international gastroenterology experts from The Chinese University of Hong Kong Medical School and Chinese Academy of Engineering Academician Shen Zuyao, endoscopy authority Professor Zhao Weiren, Professor Wang Yixiang, Professor Gao Hao, etc., published a research paper titled “Endoscopy-assisted magnetic navigation of biohybrid soft microrobots with rapid endoluminal delivery and imaging” in Science Robotics on March 17. The first authors are Wang Ben (currently an assistant professor at Shenzhen University) and Chen Qifeng, with Zhang Li as the corresponding author.
(Source: Science Robotics Official Website)
This work reports on a soft magnetic stem cell ball microrobot, abbreviated as MSCSM. To achieve the delivery of microrobots in narrow lumens within the body, the team developed an integrated robotic platform called the Endoscopy-assisted Magnetic Actuation Dual Imaging System (EMADIS). EMADIS includes a magnetic driving unit, an endoscope unit equipped with a catheter, and an imaging device. It can be used for the delivery of cell robots in various areas of the digestive system lumen, including some narrow lumens that existing medical technologies find difficult to reach, and the delivery process can be tracked in real-time step-by-step through endoscopy and ultrasound imaging (Figure 3).
Figure 3. Illustration of the soft robot’s long-distance high-precision delivery strategy based on endoscopy + magnetic control unit + ultrasound imaging.
MSCSM is primarily composed of stem cells and exhibits high activity and biocompatibility. They can be easily prepared by co-culturing stem cells and magnetic iron particles on a non-sticky surface. The stem cells and magnetic particles spontaneously assemble to form spherical biohybrid microsubstances. The size of MSCSM can also be adjusted by varying the cell dosage (adjustable range from approximately 100μm to 1 mm) (Figure 4).
Figure 4. Preparation and characterization of the soft magnetic stem cell ball microrobots. (a) Schematic diagram of self-assembly preparation of MSCSM. (b) SEM image of MSCSM. (c) SEM image of the interior of MSCSM. (d) – (f) Size regulation of MSCSM. (G) Stem cell activity within MSCSMs.
MSCSM also possesses excellent elasticity and softness. It can adapt to the complex microenvironment within the body without causing harm to the tissues/organs it passes through. For example, MSCSM can pass through much smaller gaps by deforming. After passing through the gap, it can restore its shape (Figure 5).
Figure 5. Mechanical performance of MSCSMs. (a) Schematic diagram of MSCSM passing through a narrow tube by deformation. (b) Schematic diagram and its (d) modulus changes of pressing down in a rheometer and rotating at an output frequency. (c) Optical photographs of cell balls being compressed and restored. (e) Elastic modulus data table for different human tissues. (f) Optical photographs of MSCSM passing through narrow apertures of different widths.
By applying an external magnetic field, MSCSM can perform controllable high-precision driving on various surfaces, and MSCSMs can also gather in groups and move with high precision through collective behavior. Since the stem cells on MSCSM are alive, when the applied magnetic field is anchored, they can spontaneously diffuse and proliferate on suitable surfaces, transitioning from a 3D to a 2D spreading state (Figure 6).
Figure 6. Controllable movement and spreading of MSCSMs on different surfaces. (a,b) Schematic diagram and fluorescence photographs of the process of MSCSM being released through a catheter, magnetic control movement, anchoring, and spreading. (c)-(g) Driving of MSCSM on different surfaces. (h,i) Spreading of MSCSMs on petri dishes and on 3T3 cell surfaces.
The bile duct is a typical narrow lumen within the human body. The team targeted the bile duct and conducted remote delivery of MSCSMs within the digestive system of an ex vivo pig. The microrobots were first remotely deployed in a controlled and minimally invasive manner to the intestinal area near the bile duct through the endoscope. The endoscope provided a “fast track” for MSCSM to avoid direct contact with the complex fluid environment and helped quickly traverse various biological barriers within the organs and tissues. Subsequently, the driving of the microrobots towards the entrance of the bile duct in the intestine relied on magnetic driving tracked in real-time via the endoscopic view. The tethered endoscope has limited accessible areas within the organism, while the untethered microrobots released by the endoscope broaden the targeted delivery range of the delivery system. More importantly, when the target site is within narrow lumens that are unreachable and invisible by the endoscope, the microrobots can be further located and tracked via ultrasound imaging under magnetic field driving.
Figure 7. The process of MSCSMs being delivered remotely to the internal bile duct area through the ex vivo pig’s esophagus. The entire process is tracked through a step-by-step imaging strategy using endoscopy and ultrasound imaging.
This work also confirmed that MSCSMs could release stem cells for the repair and treatment of ulcers, and the magnetic nanoparticles used showed no significant toxicity to various organs of the organism (Figure 8).
Figure 8. MSCSMs aid in accelerating the healing of gastric ulcers in rats.
EMADIS accurately delivered MSCSM to the bile duct via the esophagus, covering a total distance of approximately 100 cm, completed within 8 minutes. EMADIS has multiple advantages. First, it allows MSCSM to be rapidly deployed across multiple organ/tissue barriers throughout the body and delivered with high precision to narrow lumens that are unreachable or invisible by traditional endoscopes and medical robots. Additionally, MSCSM has low modulus, high elasticity, and high activity, and can adapt to complex environmental changes within the body through structural deformation. Finally, the natural camouflage of stem cells on MSCSMs has the potential to effectively suppress immune responses.
With the continuous development of traditional large robots and micro-robot technologies, we foresee that the integration of these two aspects will lead to the development of new delivery systems for minimally invasive medical tasks, broadening the accessibility of current clinical robotic technologies, improving delivery precision, and saving delivery and treatment time.
Paper Link:
https://robotics.sciencemag.org/content/6/52/eabd2813
Recent Progress:
– A strategy for real-time motion control and positioning of nanoparticle clusters in flowing blood environments under Doppler ultrasound guidance was proposed. (Science Advances, 2021, 7, 9, eabe5914.)
– A summary of the design, functionalization, driving control, and imaging tracking of micro-nano robots in specific biomedical issues in recent years. (Advanced Materials, 2021, 33, 2002047)
– Flexible nanomotors were found to execute fluid capture through a swinging mode. (ACS Nano, 2021, DOI: 10.1021/acsnano.0c10269)
– Nanocatalyst clusters were used for targeted removal of biofilm obstructions. (ACS Nano, 2021, 10.1021/acsnano.0c10010)
– A systematic summary of unit interactions during cluster control processes, interactions between clusters and environments, and targeted delivery strategies under positioning and imaging guidance of clusters. (ACS Nano, 2021, 15, 149)
– By controlling the different behaviors of nickel nanorods, independent pattern generation of various micro-robot swarms can be achieved in the same environment. (ACS Nano, 2021, DOI: 10.1021/acsnano.0c08284)
– A review of the motion control principles of magnetic microrobots from individuals to groups. (Annual Review of Control, Robotics, and Autonomous Systems, 2021, 1.1-1.26)
This article was submitted by the authors.
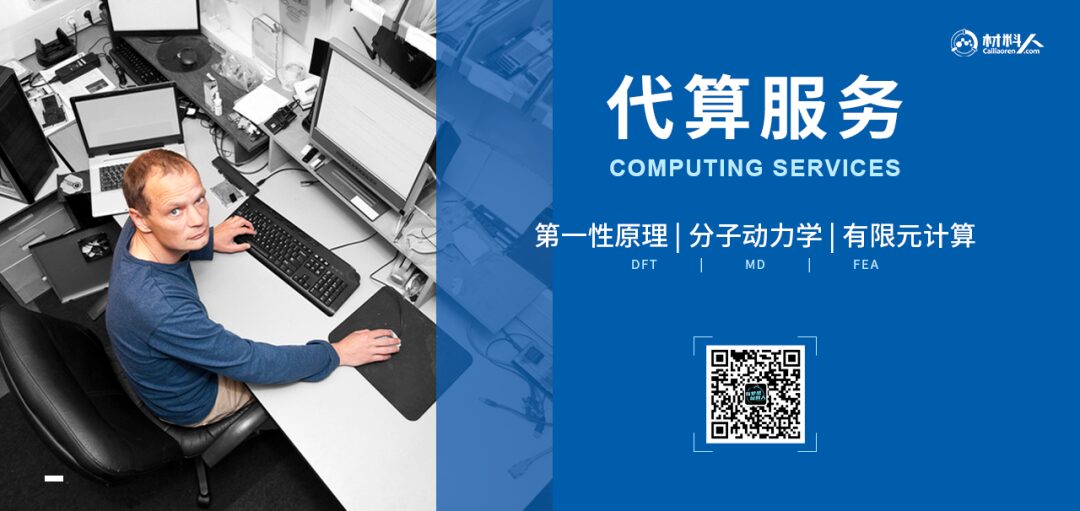
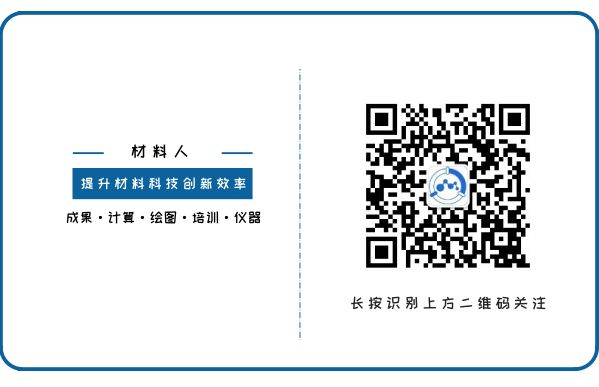