Author:Team of Zhang Jianan
First Author: Xia Huicong
Corresponding Authors: Xia Huicong Zhang Jianan
Affiliation: Zhengzhou University
Paper DOI: 10.1002/adfm.20241349
1、 Overview: This article delves into the potential applications of Electron Spin Polarization (ESP) in rechargeable batteries, analyzing how ESP can optimize battery charging and discharging efficiency, energy density, and cycle life by manipulating the direction of electron spins. The article reviews the theoretical foundations of ESP, combined with experimental techniques, showcasing the prospects and practical challenges of this technology in different battery systems.
2、 Background: With the acceleration of global electrification and renewable energy applications, the demand for high-performance rechargeable batteries is increasing. Enhancing battery energy density, charging and discharging speeds, and cycle life are key goals in today’s energy storage technology development. Electron Spin Polarization (ESP) is a quantum mechanical phenomenon that improves battery performance by controlling the direction of electron spins. In recent years, ESP has garnered widespread attention for its application in rechargeable batteries, with research indicating that this technology has the potential to greatly enhance battery energy density, charging and discharging efficiency, and cycle life. The core of ESP lies in optimizing the charge transfer process in electrode materials by adjusting the spin states of electrons. Through precise control of spins, researchers can improve battery conductivity, accelerate the transport of lithium ions, sodium ions, and potassium ions, and reduce energy loss, achieving rapid charging and extending battery life.
Key advantages of ESP technology:
Enhanced energy density: By adjusting the electron spin states, the charge transfer pathways can be optimized, significantly improving the battery’s energy storage capacity.
Accelerated charging speed: ESP technology can reduce resistance during electron conduction, thereby speeding up charging and shortening device charging times.
Extended cycle life: By suppressing side reactions that lead to battery capacity degradation, ESP helps slow down material degradation and extend battery life.
The fundamental principles of ESP in rechargeable batteries
Electron Spin Polarization (ESP) is a fundamental phenomenon affecting material magnetism, spintronics, and magnetic data storage across multiple fields. Scientists study and characterize ESP through various experimental techniques and theoretical models. These techniques include Mössbauer spectroscopy, vibrating sample magnetometry (VSM), electron paramagnetic resonance (EPR) spectroscopy, metal L-edge X-ray absorption spectroscopy, and theoretical calculations, which can profoundly reveal the complex mechanisms of ESP. For example, Mössbauer spectroscopy can provide detailed information about ESP through the interaction between nuclear spins and electron spins, while EPR and VSM are used to analyze magnetic properties of materials under different magnetic field strengths. Through theoretical calculations, researchers can simulate the influence of magnetic fields on electron spins, providing theoretical support for experiments. These methods help scientists understand the fundamental mechanisms of ESP and expand its applications in materials science, spintronics, and magnetic storage. Additionally, various strategies for controlling ESP have been developed, such as magnetic field regulation, spin injection, spin-orbit coupling, spin exchange interactions, and spin resonance. These strategies not only enhance our understanding of ESP but also promote its practical applications in modern technology. For instance, by applying an external magnetic field or introducing current, ESP can be activated and controlled, thereby improving battery efficiency and enhancing catalytic reactions.
Illustration: The main types of Electron Spin Polarization
Characteristics and Regulation Strategies of ESP
Electron Spin Polarization (ESP) plays a significant role in various fields, including material magnetism, spintronics, and magnetic storage. To unravel the complexity of ESP, scientists employ various experimental techniques and theoretical models, including Mössbauer spectroscopy, vibrating sample magnetometry (VSM), electron paramagnetic resonance spectroscopy (EPR), and metal L-edge X-ray absorption spectroscopy, combined with theoretical calculations for in-depth exploration.
1. Mössbauer spectroscopy analyzes the nuclear energy level splitting in materials by detecting the interactions between nuclear spins and electron spins, revealing the ESP phenomenon. This technique can differentiate and quantify various iron species in samples and evaluate their electron spin behavior under different environmental conditions.
2. Vibrating sample magnetometry is used to study the magnetic response of materials under an external magnetic field. It records the magnetization strength of materials through mechanical vibrations, allowing analysis of spin polarization characteristics of samples under different magnetic field strengths. Researchers have also explored the temperature-dependent magnetization curves (M-T curves) to investigate the influence of different Fe-Co ratios on spin polarization under varying temperatures.
3. EPR spectroscopy measures the absorption and emission of paramagnetic materials under microwave radiation, capable of resolving the energy level structure and dynamic behavior of electron spins in materials by adjusting magnetic field strength and frequency. EPR results show the influence of Fe-Co ratios on the degree of material spin polarization, which increases with higher iron content.
4. Metal L-edge X-ray absorption spectroscopy is used to probe the electronic structure and ESP in metals, analyzing the absorption spectra of L-edge electrons through high-energy X-rays to obtain detailed information about electron spin and orbital configurations. For example, Co L-edge spectra reveal the characteristics of unoccupied electron orbitals in Co, and analyzing the intensity ratio of L3/L2 edges further elucidates the low-spin state of Co.
5. Theoretical calculations simulate the impact of magnetic fields on electron spins using quantum mechanics and density functional theory (DFT), predicting effective magnetic moments. Studies have shown that the magnetic moments of pyridine nitrogen positively correlate with increasing iron content, and this change has been further validated in relation to catalytic activity.
These methods provide multiple avenues for characterizing ESP, deepening the understanding of its mechanisms, and expanding its applications in materials science, magnetic storage, and spintronics.
Control strategies for ESP are crucial for manipulating spin magnetic moments and have significant practical applications. The main control strategies include:
1. Magnetic field regulation: Adjusting the direction and strength of spins through external magnetic fields is widely applied in magnetic storage and magnetic resonance imaging. For instance, in magnetic storage technology, data encoding and reading can be achieved by adjusting the spin direction of magnetic materials. Moreover, studies have shown that incorporating manganese into CsPbBr₃ nanosheets and applying a magnetic field can significantly enhance the efficiency of photocatalytic carbon dioxide reduction.
2. Spin injection: By introducing current or light to excite spin polarization in materials, spin injection methods are essential in spintronic devices, allowing for precise transfer and manipulation of spins through spin-polarized currents. This method is also used in lithium-rich positive electrode materials, reducing voltage hysteresis by modulating the anionic redox reactions.
3. Spin-orbit coupling: This phenomenon is a mechanism for the conversion between spin and orbital angular momentum, which can be regulated by the crystal structure, symmetry, and electric field parameters of materials. In catalytic reactions, spin-orbit coupling can optimize electronic structures and enhance catalytic performance.
4. Spin exchange interactions: These interactions determine the dynamics of spin transfer and propagation within materials. By adjusting spin exchange dynamics, the capacity for spin information processing can be enhanced. For example, introducing superexchange interactions in Fe0.5Co0.5-MOF can accelerate the spin-dependent reaction kinetics of the oxygen evolution reaction.
5. Spin resonance: Spin resonance techniques, including nuclear magnetic resonance (NMR) and electron paramagnetic resonance (EPR), can precisely control ESP. NMR analyzes material structures by combining nuclear spins with external magnetic fields, while EPR detects the electron spin characteristics in paramagnetic materials.
These strategies not only deepen the understanding of ESP but also enhance its practical applications across multiple scientific fields, indicating that the control of spin phenomena has broad implications for modern technology and scientific advancement.
Figure 1. Characterization of Electron Spin Polarization
Figure 2. Control Strategies for Electron Spin Polarization
ESP Strategies in Rechargeable Battery Materials
Electron Spin Polarization (ESP) has been effectively induced and controlled in rechargeable batteries through various means, primarily involving magnetic materials, external magnetic fields, spin-polarized electrolytes, and advanced spectroscopic techniques. Through these methods, ESP can regulate the charge transfer processes within electrodes, significantly enhancing battery performance.
1. Magnetic materials: Magnetic materials, especially ferromagnetic elements such as iron (Fe), cobalt (Co), and nickel (Ni), can regulate ESP in battery electrodes through their net magnetic moments formed by spin alignment, thereby improving charge transfer dynamics. For example, applying magnetic films to battery electrodes can enhance their magnetic properties and increase charge transfer rates.
2. External magnetic fields: External magnetic fields also play a crucial role in controlling ESP in batteries. Aligning ESP along predetermined directions through magnetic fields can significantly enhance charge transfer efficiency. Research indicates that pulsed magnetic fields help improve the capacity and cycle life of lithium-ion batteries (LIBs).
3. Spin-polarized electrolytes: The use of spin-polarized electrolytes further expands the control dimensions of ESP. In these systems, the ions in the electrolytes exhibit preferred spin orientations, directly affecting the charge transfer dynamics at the electrode-electrolyte interfaces. Optimizing the ESP of electrolytes can significantly enhance charge transfer efficiency and the overall energy storage capacity of batteries.
4. Advanced spectroscopic techniques: Techniques like electron spin resonance (ESR) spectroscopy are crucial for characterizing and quantifying ESP in battery systems. ESR spectroscopy provides detailed information about the characteristics of ESP in battery materials, revealing the mechanisms driving charge transfer processes. Combining ESR with X-ray photoelectron spectroscopy (XPS) and transmission electron microscopy (TEM) tools can provide a more comprehensive understanding of the interactions between ESP and battery performance.
Additionally, researchers have explored materials and structures with inherent ESP characteristics, including transition metal oxides, magnetic nanoparticles, and organic radicals. These materials have the potential for application in rechargeable batteries as electrodes, electrolytes, or performance-enhancing additives. For example, transition metal oxides such as LiCoO2, LiNiO2, and LiMn2O4 exhibit significant ESP characteristics due to their unique electronic structures and magnetic properties, and their ESP properties can be further optimized through doping and valence state adjustment to enhance battery performance. Magnetic nanoparticles like Fe3O4 and CoO, owing to their inherent magnetic properties, can facilitate efficient charge transfer and energy storage. Through functionalization, these nanoparticles are considered strong candidates for next-generation battery technologies. Organic radicals, such as nitrogen oxides, have been explored as electrolyte additives that can induce ESP, optimize charge transfer dynamics, and enhance battery performance.
ESP significantly influences the performance of rechargeable batteries and is deeply affected by material and structural configurations. However, increasing research indicates that optimizing ESP can significantly enhance multiple performance metrics of batteries. By optimizing ESP within electrodes, battery capacity can be increased, charging and discharging rates accelerated, side reactions minimized, and cycle life extended. For example, introducing organic radicals into electrolytes has been shown to reduce degradation in positive electrodes, thereby prolonging battery life. Furthermore, ESP can facilitate the integration of renewable energy with the grid, enhancing energy storage and conversion efficiency.
In summary, ESP offers a powerful research direction for enhancing rechargeable battery technology. By leveraging the unique characteristics of electron spins, researchers can optimize charge transfer processes within battery electrodes. Despite facing some practical implementation challenges, ESP still exhibits tremendous potential benefits. As research in this field continues to deepen, ESP is expected to play a key role in developing more efficient and reliable energy storage solutions, promoting the future development of sustainable energy.
Applications and Progress of ESP in Rechargeable Batteries
Electron Spin Polarization (ESP) represents a cutting-edge innovation in battery technology, enhancing performance based on the magnetism of electrons. ESP reduces the resistance of ion mobility by regulating the ion movement pathways in electrode materials, improving conductivity, accelerating charging speeds, and extending cycle life. Research indicates that ESP can enhance battery capacity and stability by lowering the energy barriers for ion insertion and extraction, improving the cycling performance of rechargeable batteries.
Currently, the application of ESP in rechargeable batteries primarily focuses on the use of magnetic materials such as iron, cobalt, and nickel, which can enhance the charge transfer efficiency of batteries through their spin polarization characteristics. Additionally, external magnetic fields are a common method for inducing ESP. However, the feasibility of using external magnetic fields in everyday battery systems still faces challenges, and current research is mainly at the laboratory stage, but its potential advantages make it a hotspot for future research.
1) Lithium-ion batteries (LIBs)
Lithium-ion batteries (LIBs) are fundamental to portable electronic devices and electric vehicles, but their performance is limited by the diffusion rates of lithium ions and the electron transfer speeds in electrode materials. By regulating the electron spin states in electrodes through ESP, conductivity and lithium ion transport rates can be significantly improved, reducing the energy barriers for lithium ion migration, thereby accelerating battery charging and discharging speeds, increasing capacity, and extending cycle life.
Research shows that the energy storage capacity of transition metal oxide electrodes exceeds expectations, primarily attributed to the strong surface capacitive effects generated by ESP. Additionally, magnetic nanoparticles and transition metal fluorides, such as FeF2, can further enhance charge transfer capabilities through ESP.
2) Sodium-ion batteries (SIBs)
Sodium-ion batteries (SIBs) are gaining attention as substitutes for lithium-ion batteries due to the abundance and lower cost of sodium resources. The application of ESP in SIBs can optimize charge transfer dynamics in electrode materials through magnetic interactions, enhancing the diffusion rates of sodium ions, thereby improving battery performance. Research indicates that using Fe3O4 coatings to enhance ion diffusion speed and uniformity at the electrode interface can significantly improve the overall performance of sodium-ion batteries.
3) Potassium-ion batteries (PIBs)
Potassium-ion batteries (PIBs) have potential for large-scale energy storage applications due to the abundance and low cost of potassium resources. However, PIBs still face challenges in energy density and charging/discharging rates. ESP can improve the transport efficiency of potassium ions, reducing charge transfer resistance, further enhancing the electrochemical performance of PIBs. Moreover, ESP can also stabilize the spin environment of electrode materials, reducing side reactions with electrolytes, thereby extending battery life.
4) Lithium-sulfur batteries (LSBs)
Lithium-sulfur batteries (LSBs) are attracting attention due to their high theoretical energy density, but their practical applications are limited by the poor conductivity of sulfur and the dissolution of lithium polysulfides (LiPS) in electrolytes. ESP strategies can enhance electronic conductivity and promote more efficient redox reactions by regulating the electron spin states at the electrode interfaces. Utilizing ESP can enhance the binding of sulfur-based materials with LiPS, suppressing their dissolution and significantly improving the cycling stability of lithium-sulfur batteries.
In lithium-sulfur batteries, research indicates that ESP can enhance the catalytic performance of electrode materials by optimizing spin density, significantly improving the kinetics of lithium-sulfur redox reactions and enhancing charging and discharging performance. Furthermore, ESP can facilitate the reduction of Li2S2 to Li2S in single-atom catalysts, further extending battery life.
In summary, ESP holds tremendous potential for the development of rechargeable battery technology. By precisely regulating the spin characteristics of electrode materials, ESP can enhance battery energy density, charging and discharging rates, and cycle life. As research on ESP continues to deepen, it is expected to lead to the development of more efficient and reliable energy storage solutions, advancing sustainable energy development.
Figure 3. Schematic of spin-polarized electrons in electrochemical systems and magnetometer analysis
Figure 4. Mechanisms and spin polarization responses of high-pressure LiCoO2 and transition metal particle electrodes
Figure 5. Spin polarization of Na+ diffusion and structural analysis in sodium-ion batteries
Figure 6. Spin transitions and reversible charging/discharging mechanisms in iron and MoS2 electrodes
Figure 7. Spin polarization of Co-doped iron cyanide in potassium-ion batteries, K+ adsorption, and electrochemical processes
Figure 8. Electron spin polarization for the conversion of LiPS and ion transport in sulfur-based batteries
5. Conclusions and Outlook
This comprehensive analysis of Electron Spin Polarization (ESP) in the applications of rechargeable batteries reveals its significant potential in the energy storage field, summarizing the following key findings:
ESP influences charge transfer and redox reactions in electrode materials: By adjusting the spin characteristics of materials, researchers can precisely control electron flow, significantly enhancing the electrochemical performance of batteries. This lays the groundwork for integrating ESP into battery systems.
Challenges faced: Despite the broad application prospects of ESP, challenges remain regarding the scalability of material synthesis, operational stability, integration with existing manufacturing processes, and safety. Addressing these issues requires targeted research to promote the practical application of spin-polarized materials in battery technology.
Material design and engineering strategies: To overcome the aforementioned challenges, researchers are developing advanced material design and engineering strategies, including innovative synthesis techniques and the introduction of protective layers. Additionally, efforts to align these materials with traditional manufacturing processes and promote collaboration and standardization are crucial for the dissemination of spin-polarized materials.
Multiple advantages of ESP: ESP technology is expected to enhance battery storage capacity, accelerate charging speeds, and extend battery cycle life. These improvements are crucial for large-scale energy storage systems and contribute to the development of sustainable, clean energy solutions. The widespread application and commercialization of ESP technology are anticipated to have a profound impact on the global energy landscape.
Future outlook: The prospects of ESP in energy storage technology are exciting. Researchers are working to develop the next generation of batteries with unprecedented energy densities, rapid charging capabilities, and longer lifetimes. These technologies will power applications in consumer electronics, electric vehicles, and grid-scale energy storage systems. The application of ESP technology can not only promote the achievement of global sustainable development goals but also facilitate the large-scale adoption of renewable energy, reducing reliance on fossil fuels.
Economic and social impacts: The commercialization of ESP technology is expected to stimulate economic growth and drive transformation across multiple industries. With the increasing demand for high-performance energy storage solutions, ESP technology will create opportunities in sectors such as transportation, communications, and renewable energy, enhancing job opportunities and strengthening the development of the energy storage industry.
The importance of interdisciplinary collaboration: The advancement of ESP technology relies on collaborative efforts across multiple fields, including electrochemistry, nanotechnology, and materials science. Cooperation among academia, industry, and regulatory bodies will accelerate innovation while ensuring the establishment of safety standards, regulations, and best practices to promote the sustainable development of spin-polarized batteries.
In summary, ESP technology holds great promise in the energy storage field and has the potential to transform the global energy landscape. By addressing challenges and seizing opportunities, ESP will make significant contributions to a cleaner, more efficient, and interconnected world.
Author Introduction:
Xia Huicong, researcher at the School of Materials Science and Engineering, Zhengzhou University. PhD from Zhengzhou University in 2022 (Supervisor: Professor Zhang Jianan), joint training at the Dalian Institute of Chemical Physics, Chinese Academy of Sciences from 2019-2020 (Co-supervisor: Researcher Deng Dehui), Postdoctoral fellow at Zhengzhou University in 2023 (Co-supervisor: Professor Shan Chongxin). Main research directions include the design, preparation, and energy storage mechanism studies of carbon-based multifunctional electrode materials in lithium/sodium-ion batteries. Over 20 SCI papers published as the first author and corresponding author in journals such as Angew. Chem., Energy Environ. Sci., Adv Energy Mater. Serving as editorial board member and guest editor for several journals including Exploration, Chinese Chemical Letters, Energy Lab, Carbon Neutralization, Journal of Central South University.
Zhang Jianan, professor at the School of Materials Science and Engineering, Zhengzhou University, doctoral supervisor, Young Changjiang Scholar of the Ministry of Education, Fellow of the Royal Society of Chemistry, member of the Women’s Committee of the Chinese Chemical Society, member of the Youth Committee of the Chinese Chemical Society. In recent years, he has focused on the design, construction, application, and related catalytic process mechanism research of carbon-based composite materials in proton exchange membrane fuel cells (PEMFCs) and metal-air batteries, making significant progress. Notable achievements include developing template-free nanoconfined and thermodynamic control methods to achieve carbon-limited multi-active center energy catalytic materials, addressing the issue of activity decay in electrode materials during electrochemical processes; revealing the synergistic catalytic mechanisms of nano-clusters and single atoms in non-precious metal-nitrogen-carbon catalysts; and utilizing theoretical calculations combined with experimental methods to reveal the relationship between the built-in micro-magnetic field environment of transition metal active centers and catalytic performance, achieving various stable and efficient new energy materials. To date, over 70 papers published in journals such as Nat. Commun., Angew Chem. Int. Ed., Adv. Mater., Energy Environ. Sci., Adv. Energy Mater., ACS Nano, Adv. Funct. Mater. Many articles have been rated as ESI highly cited articles; awarded the first prize for provincial scientific and technological achievements in 2020 (Ranked 1), and the second prize for the Henan Provincial Natural Science Award in 2021 (Ranked 1); serving as editorial board member and guest editor for several journals including Chemical Journal of Chinese University, InfoMat, Chinese Chemical Letters, Electrochemistry; also serving as a member of the Youth Committee of the Chinese Chemical Society and the Women’s Committee of the Chinese Chemical Society. Selected as one of the top 2% of global scientists in 2022.
Paper Information:
Electron Spin Polarization in Rechargeable Batteries: Theoretical Foundation and Practical Applications
Huicong Xia*, Yao Hu, Zixin Li, Haihui Lan, Jianan Zhang*
Advanced Functional Materials
DOI: 10.1002/adfm.202413491
Click the bottom left corner “Read the original text” to view the original paper.
Advanced
Functional
Materials
Journal Introduction
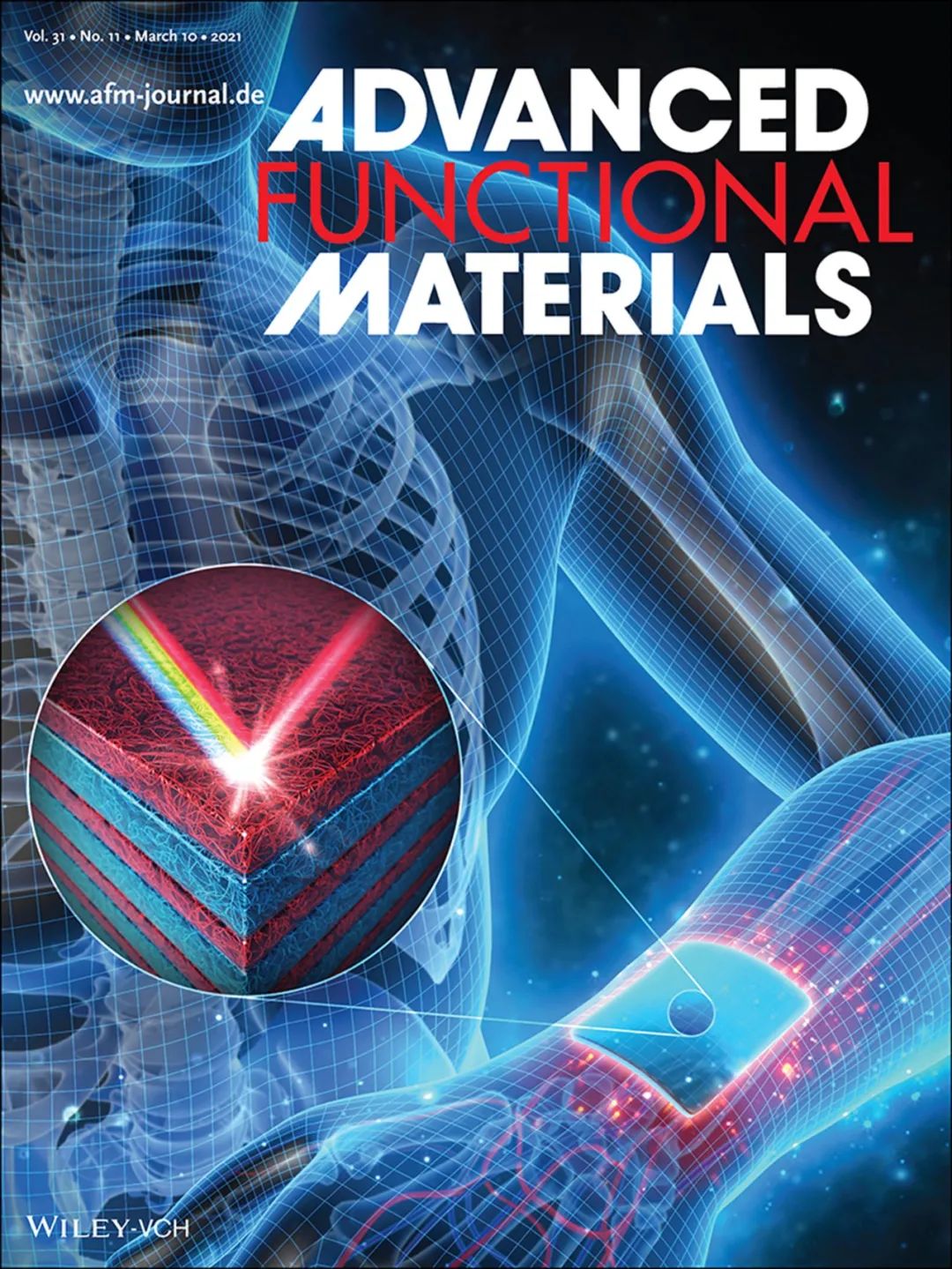
“Advanced Functional Materials” is one of the well-known materials science journals under Wiley Publishing, focusing on significant breakthroughs in materials science related to applications in nanotechnology, chemistry, physics, and biology.