In inductively coupled plasma mass spectrometry (ICP-MS), atomization and ionization are achieved in a radiofrequency argon plasma at atmospheric pressure.
Since a seminal publication in 1980, ICP-MS has become one of the most frequently employed methods of elemental MS.
The wide acceptance of ICP-MS is due to its comparatively robust, yet versatile sampling mode.
An ICP not only offers high ionization efficiency for elements of low IE, e.g., 98% for IE = 7 eV, it is still applicable to nonmetals such as P and even Cl (ionization efficiency is 1% for IE = 13 eV).
The core of an ICP source is formed by a so-called plasma torch.
It consists of three coaxially aligned quartz tubes that are inserted along the central axis of a water-cooled RF coil.
After ignition by an electric spark discharge, the coil (typically RF of 27 MHz) feeds the plasma by coupling electric energy (1-2 kW) into the gas, because the fluctuating magnetic field causes ion motion which in turn heats up the gas and maintains a continuously flowing plasma.
The outer quartz tube is about 20 mm in diameter and has its walls cooled by an argon gas flow of 12-20 l min-1.
The middle tube supplies another stream of argon, the so-called auxiliary gas flow of 1-2 l min-1 that is feeding the plasma.
The sample is then introduced into the center of the toroidal plasma by an argon carrier gas flow of 1-1.5 l min-1.
This carrier gas passes through a nebulizer and leads to the dissipation and transfer of a liquid sample as micrometer-sized droplets (depending on the type of nebulizer), which can be vaporized, atomized, and ionized within the ICP.
Typical sample consumption is in the order of 0.02-1 ml min-1.
The ICP approaches a temperature of 10,000 K in the induction zone close to the coil, in the center of which evaporation and atomization occur at about 8000 K.
While the plasma flows away from the coil, excitation of the neutrals is effected at 7500 K followed by ionization in a zone well after the coil at about 6000 K.
Thereafter, recombination in the plasma’s tail causes molecular interferences, which can be reduced by placing a collision cell in the ion beam, for example.
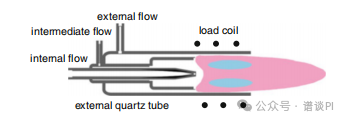
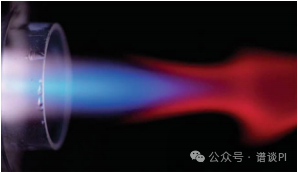
The appearance of the plasma varies and depends on liquid and gas flow, nebulizer design, and of course, the type and concentration of the analyte.
In some cases, the ICP can be extraordinarily beautiful.
Careful observation of the ICP by high-speed photography can reveal carryover of particles from laser ablation (LA) or of droplets into the ICP due to overly high liquid flow.
Analogous to ESI, ion transfer into the mass analyzer is accomplished via a differentially pumped interface.
A small portion of the plasma enters the first pumping stage through a hole in the center of the sampling cone.
Water cooling of the sampling cone preserves its surface from rapid destruction due to the exposure to the hot plasma.
Next, the ions are guided through the entrance of the skimmer by application of an electric potential, while most neutrals are pumped off the supersonic expansion in this region.
Analogous to early atmospheric pressure ion sources of organic MS, the first implementation of ICP-MS was attached to a quadrupole analyzer due to its acceptance of moderate vacuum conditions.
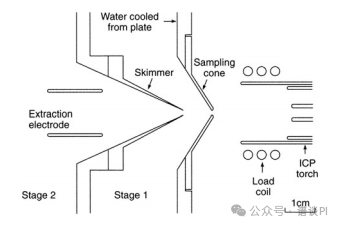
Nowadays, ICP sources are used with magnetic sector, to an important extent time-of-flight (TOF), and even Fourier transform ion cyclotron resonance (FT-ICR) mass analyzers.
The interest in high-resolution ICP instruments is driven by polyatomic interferences between the plasma gas and the matrix introduced, which are indeed limiting factors for ICP-QMS.
Isobaric interferences are less problematic than it might appear on first sight, since most of the elements have more than two isotopes to select from.
Anyway, none of the isobaric interferences can be resolved by the current sector or TOF mass spectrometers since they require a mass resolution above 12,000.
Multicollector detection systems are an important feature of element MS instrumentation as simultaneous detection of all ions of interest provides the most accurate isotope ratios.
The traditional approach employs multiple Faraday cups that can be adjusted along a sophisticated rail to collect a set of ions.
A more recent ICP mass spectrometer allows ions along the 120-mm focal plane of a small Mattauch-Herzog geometry analyzer to be detected simultaneously.
This is accomplished by use of a large direct charge semiconductor detector that provides 4800 channels covering the focal plane.
Thus, 210 nuclides from 6Li to 238U can be monitored without scanning, i.e., at constant magnetic field.
The particular ICP mass spectrometer even relies on a permanent magnet and its ion optical path is complemented by a 127° electrostatic sector as pre-filter in front of the Mattauch-Herzog analyzer in order to prevent photons and neutrals from the ICP from entering the actual mass analyzer.
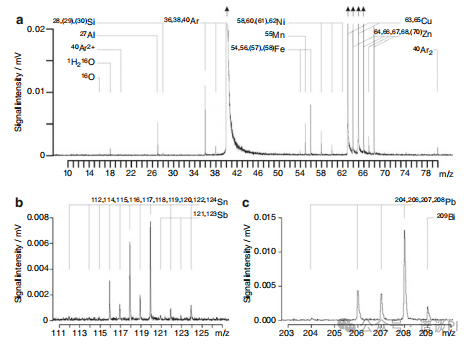
Distinguished acronyms
In inorganic mass spectrometry, the type of mass analyzer occasionally becomes part of the acronym: a quadrupole leads to ICP-QMS, a magnetic sector field leads to ICP-SFMS, and use of a double-focusing analyzer may be indicated by ICP-DFMS, respectively.
Thus, a time-of-flight analyzer attached to an ICP source would be referred to as ICP-TOFMS.
Again, this is slightly different from the customs in organic and biomedical mass spectrometry where only TOF and FT-ICR tend to be included in the acronym and normally are separated by a hyphen from MS.
Liquid sample introduction is still the standard in ICP-MS.
Consequently, the coupling of liquid chromatography or capillary electrophoresis to ICP instruments is straightforward.
This way, not only the presence of an element in a sample can be detected, but also the assignment to molecular species becomes feasible.
This explains the relevance of ICP-MS for the identification of non-C,H,N,O elements in proteins, i.e., for metallomics (cf. first two examples of this chapter).
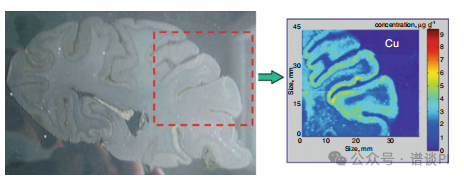
ICP-TOFMS of brassThree categories of signals are contributing to the ICP mass spectrum of brass.
The background peaks due to 14N+, 16O+, 1H2O+, 40Ar2+, 36,38,40Ar+, and 40Ar2+ are contributions of the ICP background spectrum.
Then, the polyisotopic elements Cu and Zn represent the main components of brass, and thus, cause strong peaks.
Finally, Si, Mn, Fe, Ni, Sn, Sb, Pb, and Bi are present at low concentration.
Instead of nebulizing a solution of the alloy, in this particular case the brass has been fed into the ICP by in-torch laser ablation.
Need for sample preparation
ICP-MS is commonly mentioned in the context of high throughput and claimed to have no need for extensive sample preparation.
In analytical practice, however, every sample needs to be treated individually; digestion prior to analysis usually takes about 2 h per sample.
Furthermore, as is the case with TIMS for example, matrix separation is required for precise and accurate isotope ratio determinations.
In particular, if MC-ICP-MS is going to be used as a complementary technique for TIMS, high throughput is only achieved on the expense of precision.