Click the blue text
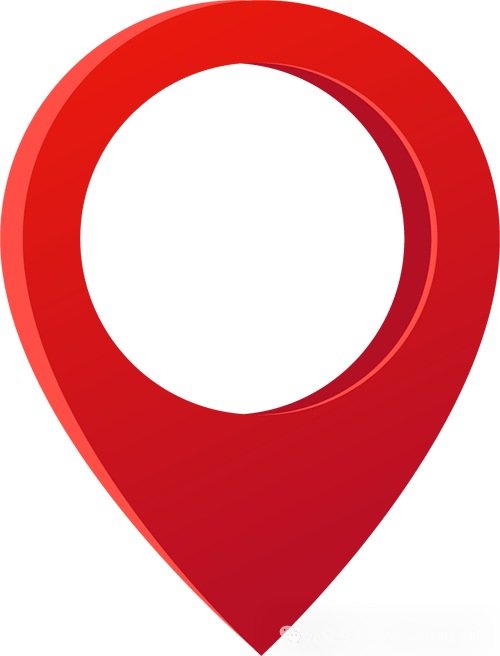
Follow us
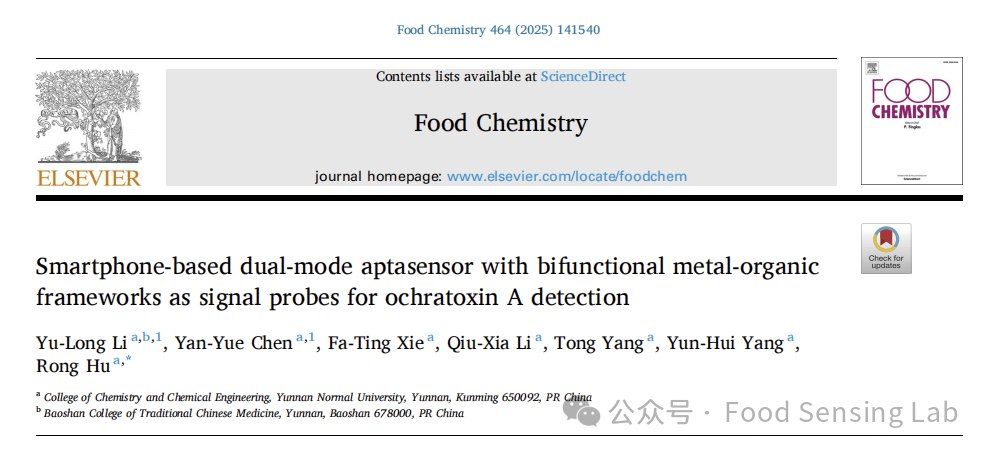
Introduction /Introduction
The POCT system integrates the electrochemical biosensor with a smartphone interface in a plug-and-play manner. This homogeneous electrochemical biosensor avoids multiple self-assembly steps and coexisting substances on the electrode surface, significantly improving hybridization efficiency and selectivity. Additionally, the operation requires only two washing steps, greatly simplifying the procedure. Furthermore, Fe-MIL-88 acts as a nanoenzyme, catalyzing TMB to oxidized TMB, resulting in a color change that is visible to the naked eye or measurable using a UV-visible spectrophotometer. Digital smartphone analysis employs a dual-mode strategy utilizing the three-color channels (red, green, and blue). By cross-validating signals and providing diverse readings, this dual-mode detection platform is expected to offer stronger anti-interference capabilities and more reliable results, significantly enhancing detection accuracy.
Related findings have been published under the title “Smartphone-based dual-mode aptasensor with bifunctional metal-organic frameworks as signal probes for ochratoxin A detection” in Food Chemistry.
Research Figure Text/Introduction
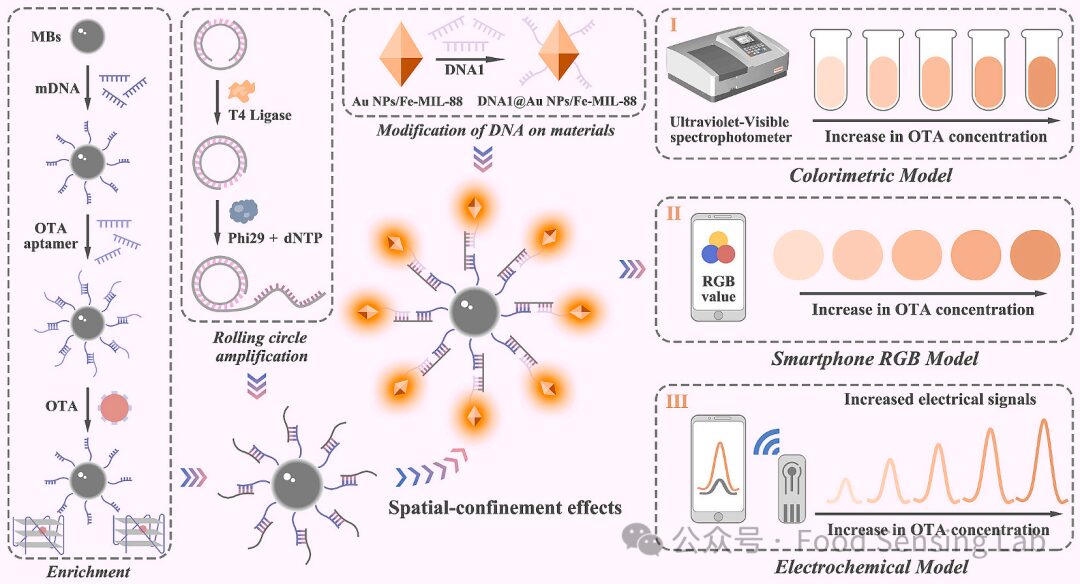
Fig 1. Schematic illustration of the dual-mode POCT platform.
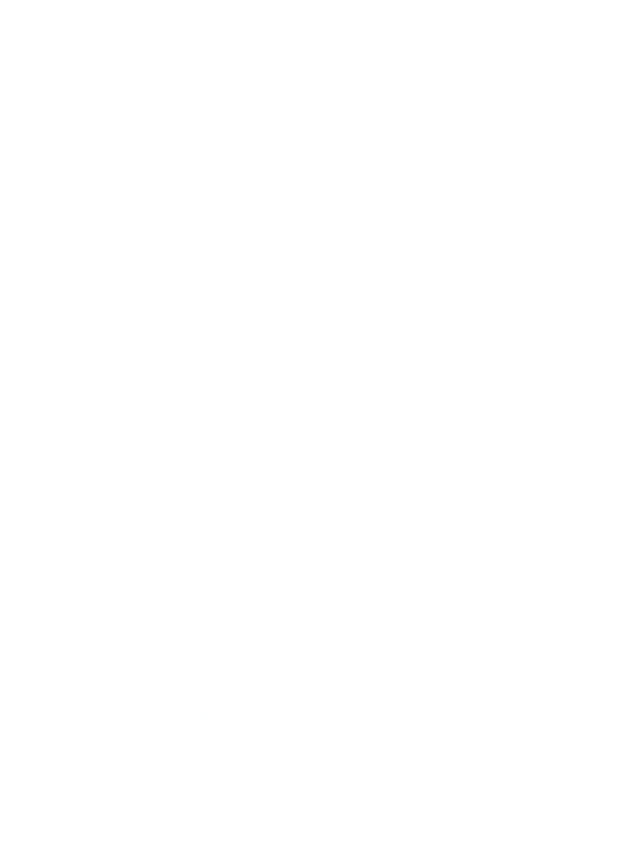
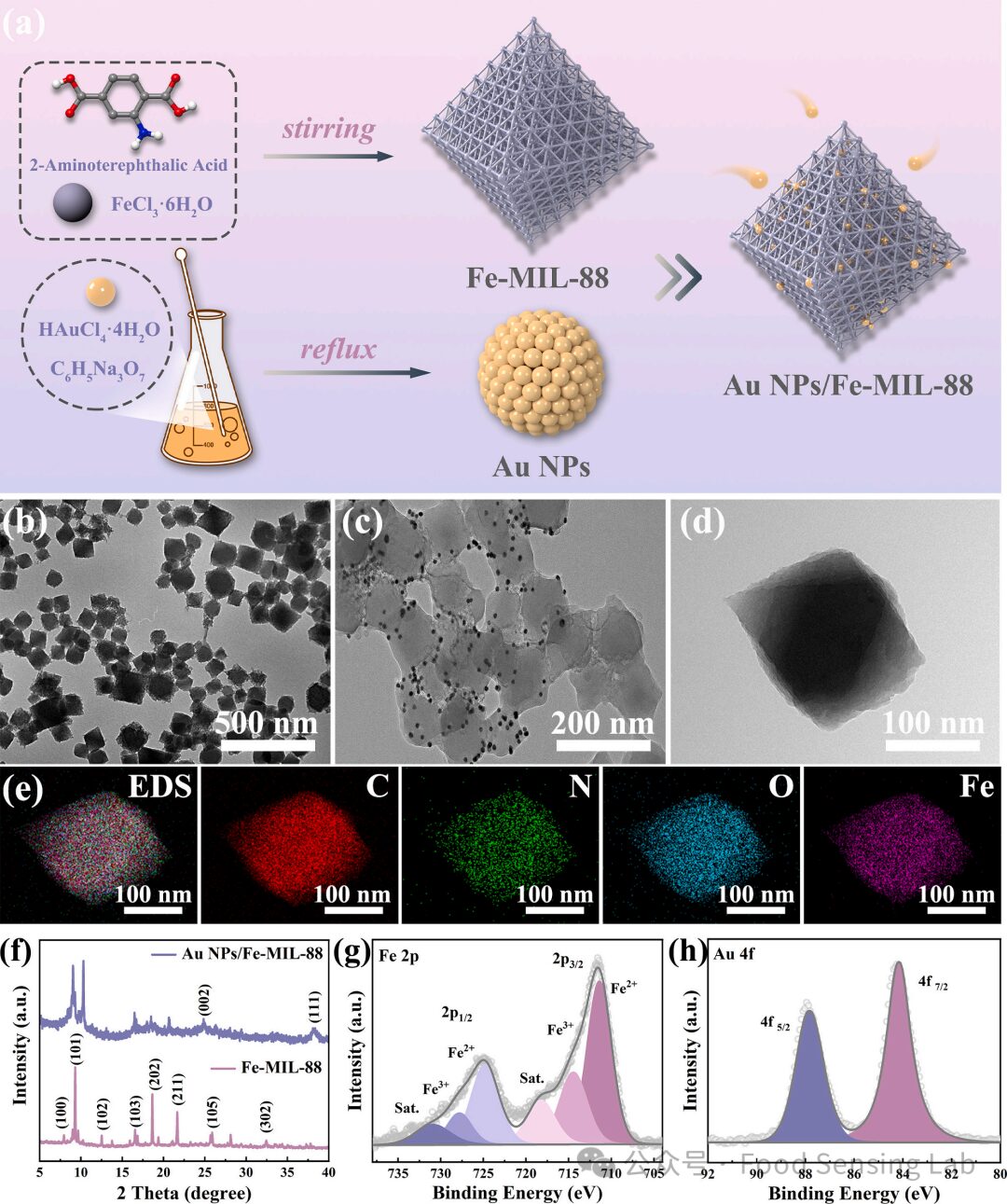
Fig 2. (a) Schematic diagram of Au NPs@Fe-MIL-88 synthesis process. TEM images of (b, d) Fe-MIL-88 and (b) Au NPs@Fe-MIL-88. (e) EDX mapping of Fe-MIL-88. (f) XRD patterns of Fe-MIL-88 and Au NPs@Fe-MIL-88. (f, g) XPS spectra of Fe 2p and Au 4f, respectively.
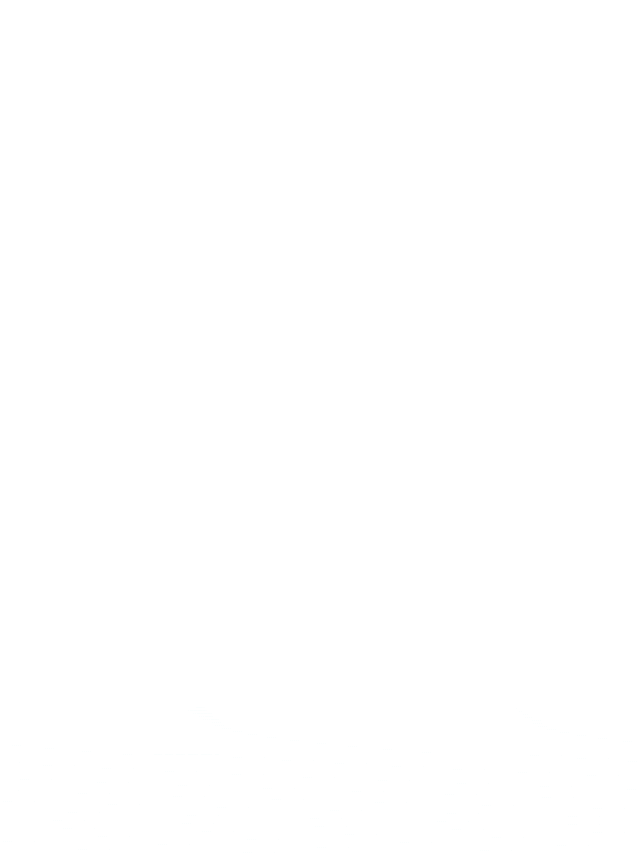
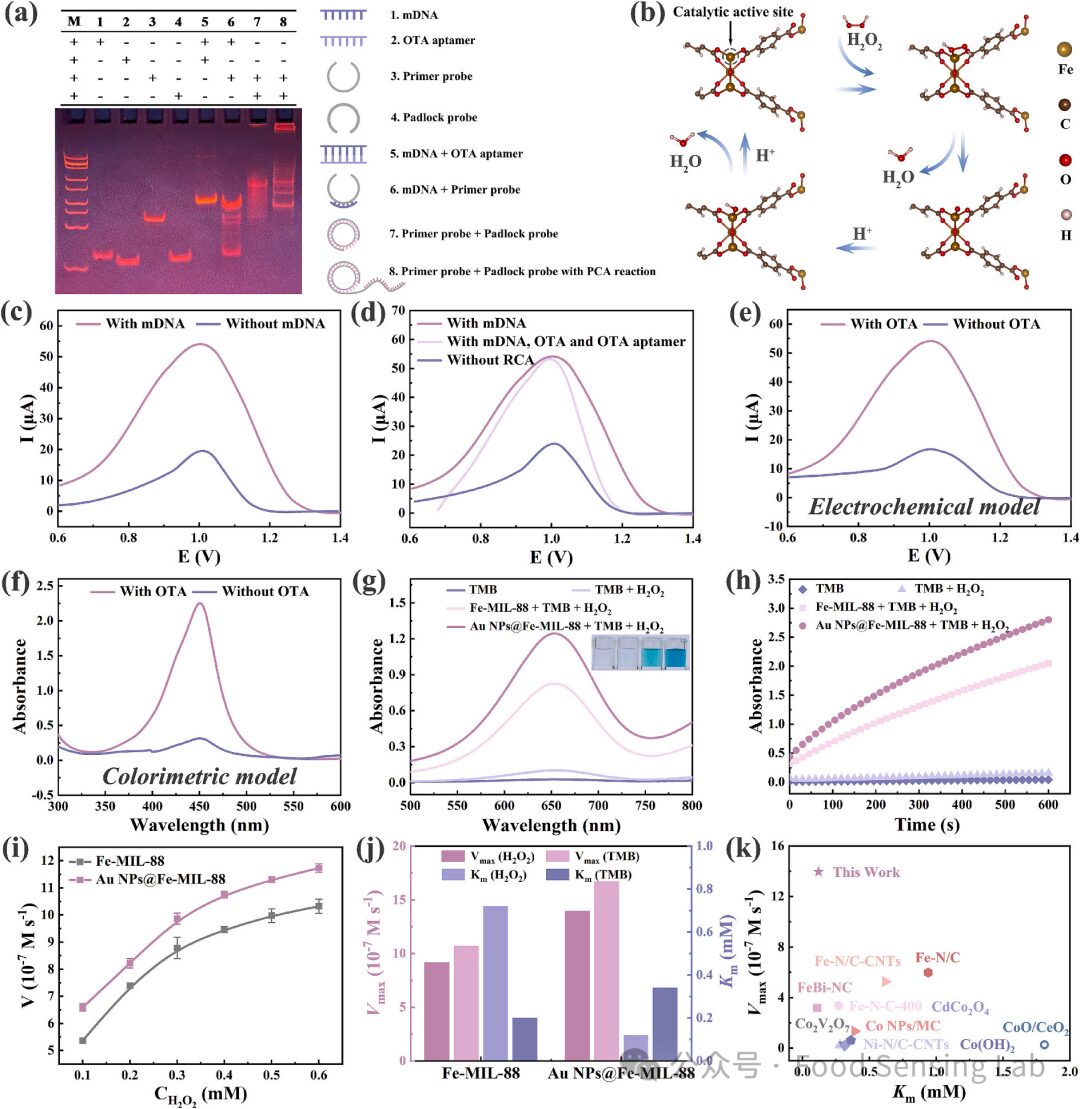
Fig 3. (a) PAGE electrophoresis. DNA molecular weight markers (band M), mDNA (band 1), OTA aptamer (band 2), primer probe (band 3), padlock probe (band 4), mDNA+OTA aptamer (band 5), mDNA+primer probe (band 6), primer probe+padlock probe (band 7), and primer probe+padlock probe with RCA reaction (band 8). (b) Reaction pathway for reducing H2O2 with optimized adsorption configurations on Fe-MIL-8. (c) DPV current response with and without mDNA. (d) DPV current response without OTA aptamer (purple curve), without RCA reaction (blue curve), and all existed (lavender curve). (e) DPV current response with and without target OTA for electrochemical mode. (f) UV–vis spectrum in the absence and presence of target OTA for colorimetric mode. (g, h) The POD activity of Fe-MIL-88 and Au NPs@Fe-MIL-88. (i, j) Steady-state kinetic of Fe-MIL-88 and Au NPs@Fe-MIL-88. (k) Comparison with other known catalysts. (For interpretation of the references to color in this figure legend, the reader is referred to the web version of this article.)
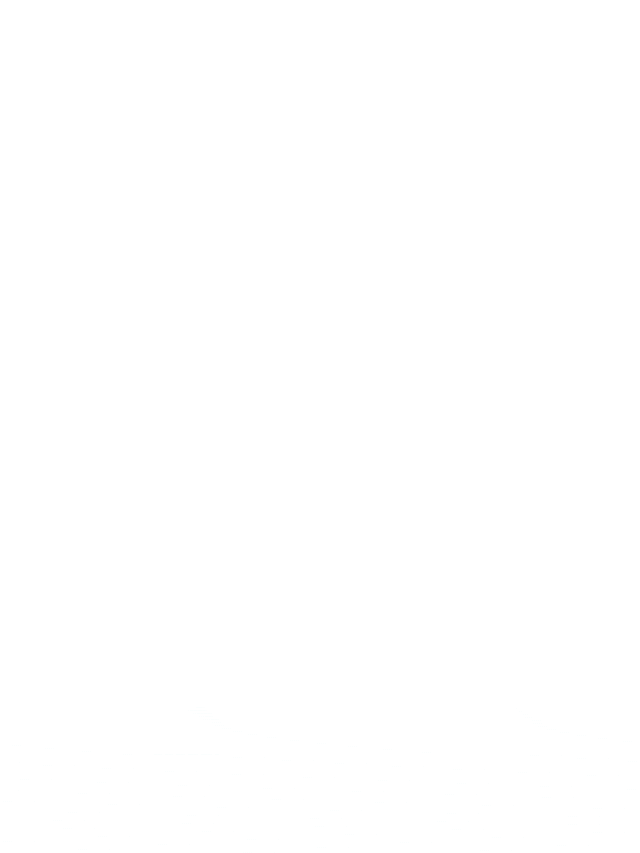
Fig 2. (A) XRD pattern of CdS/MoSe2, CdS, and MoSe2. (B) Survey and high-resolution XPS spectra of (C) Mo 3d, (D) Se 3d, (E) Cd 3d, and (F) S 2p of CdS/MoSe2.
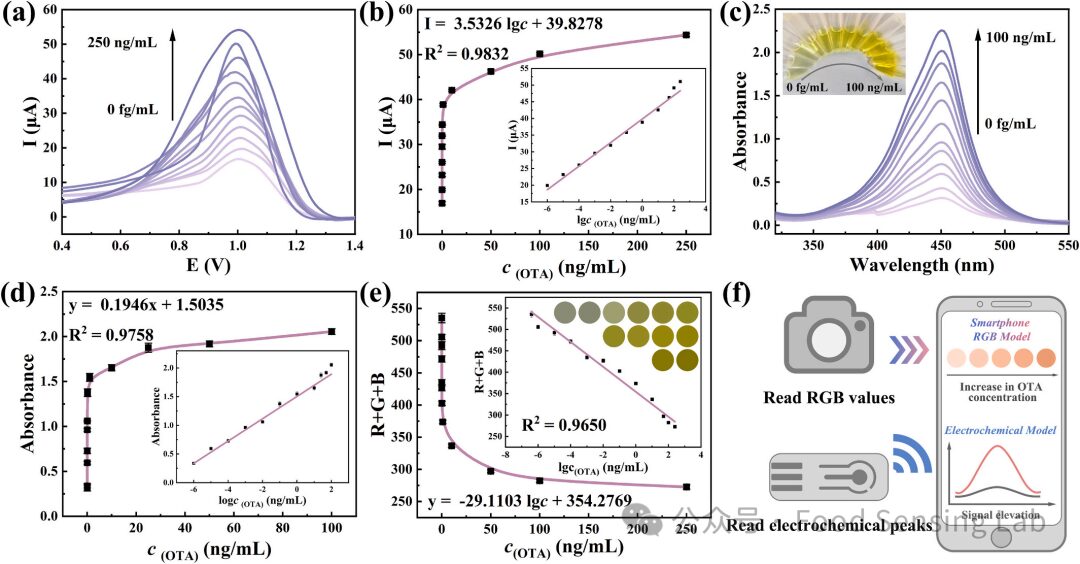
Fig 4. (a) DPV response curves of dual-modal aptasensor at varying OTA concentrations. (b) The current response increased with increasing OTA concentrations. The inset illustrates the correlation between the current response and the logarithmic values of different OTA concentrations. (c) Uv-vis spectrum at 450 nm for different OTA concentrations. (d) Absorbance intensity increased with rising OTA concentrations. The inset demonstrates the correlation between absorbance and the logarithmic values of different OTA concentrations. (e) The relationship between the RGB value and the logarithmic value of different OTA concentrations. (f) Model scheme for OTA detection using an intelligent sensing platform.
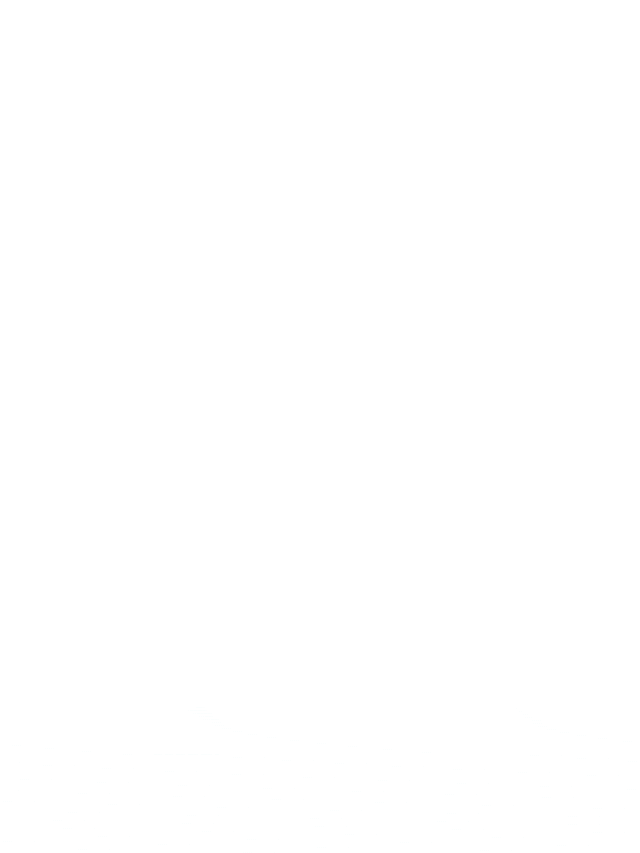
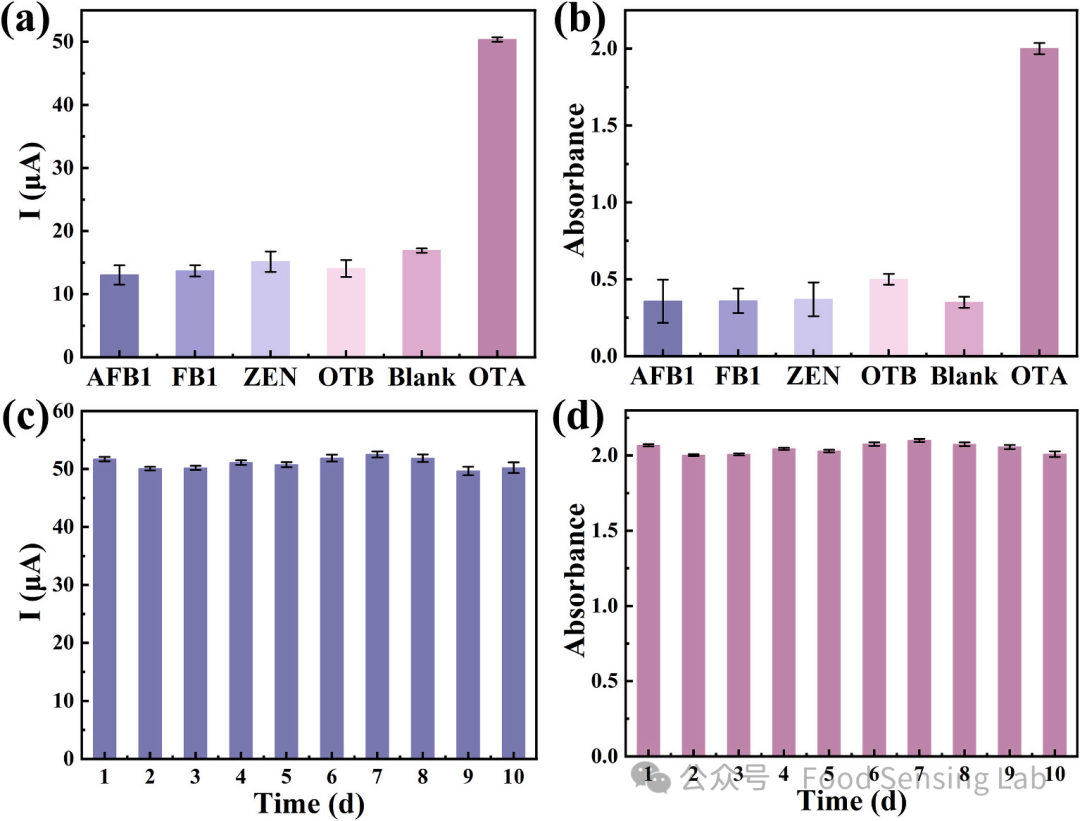
Fig 5. (a, b) The selectivity of the dual-mode aptasensor. (c, d) The reproducibility of the dual-mode aptasensor.
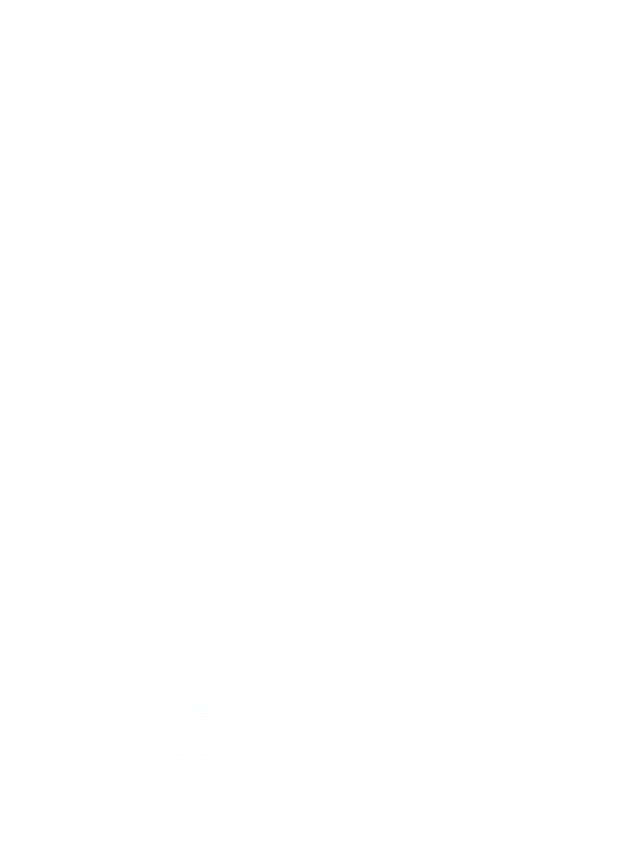
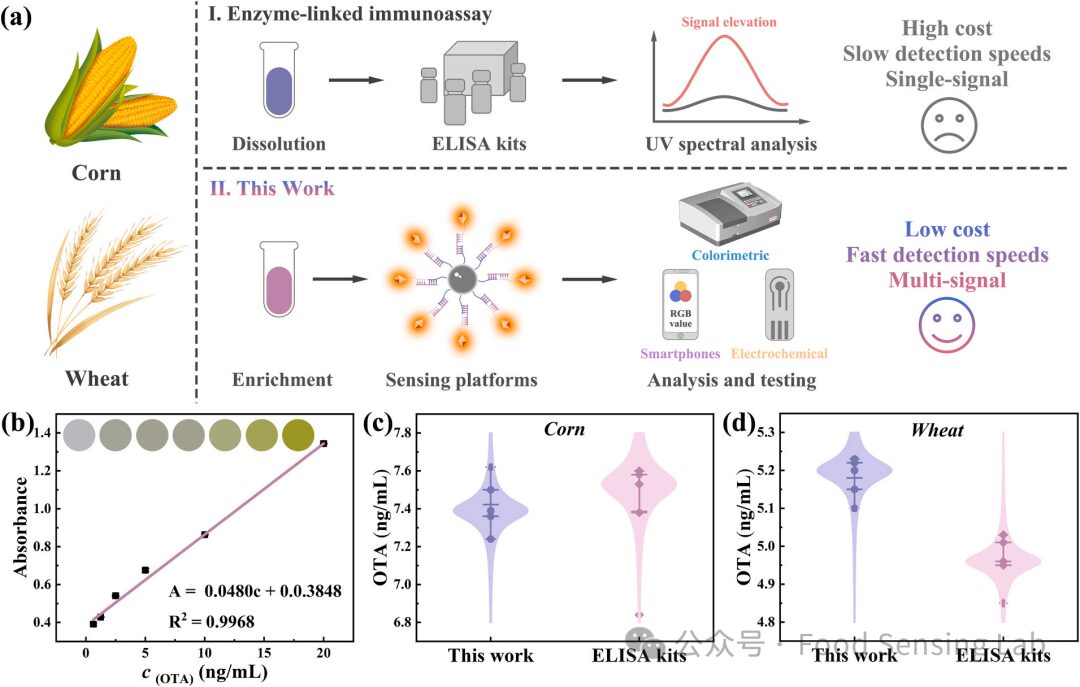
Fig 6. (a) Model scheme for the detection of OTA using ELISA kits and the proposed method. (b) Detection of OTA using commercial ELISA kits. The relationship between the absorbance value and the logarithmic value of different OTA concentrations. (c, d) The detection of real corn samples and wheat samples, respectively.
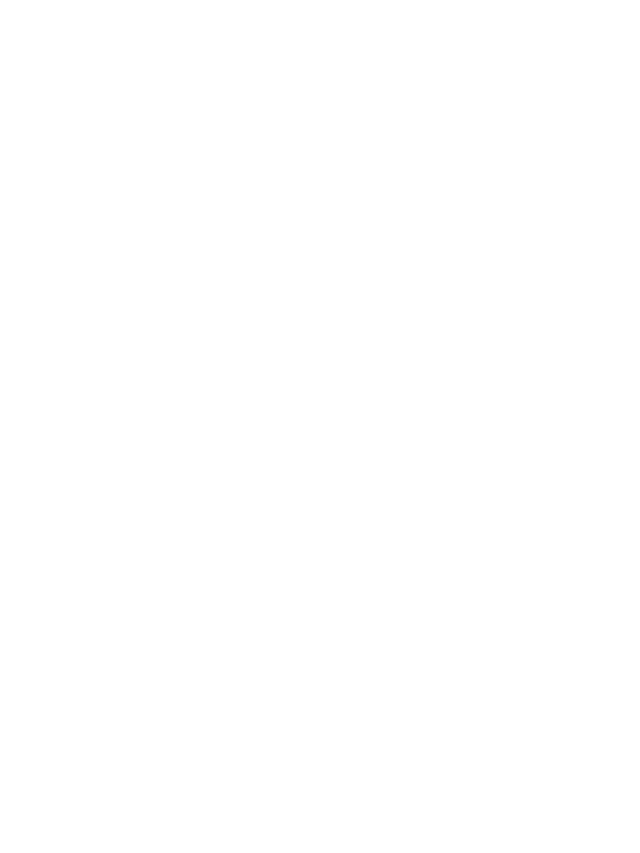
(a) Sensing response and (b) response loss curves of Sn1Co sensor towards 100 ppm H2S under various humidity at RT. Repeatability curves toward 50, 100 and 200 ppm H2S under 25 % RH at RT: (c) Sn0.25Co, (d) Sn0.5Co, (e) Sn1Co, and (f) Sn2Co sensors.tection solutions with different concentrations. (c) RGB values vs. the TA concentrations. (d) Linear plot showing the relationship between the R values and the TA concentrations. Error bars represent standard deviations of three repetitive measurements.
Conclusion /Conclusion
The team successfully constructed a colorimetric/electrochemical/smartphone dual-mode aptasensor for the high-sensitivity, easy, POC detection of OTA in food samples. In the electrochemical DPV mode, OTA detection was performed using an electrochemical POC biosensor equipped with a smartphone and a plug-and-play approach. The homogeneous reaction requires only two washing steps, significantly simplifying the operational procedure. Fe-MIL-88 exhibits catalytic activity, decomposing H2O2 into ·OH radicals, generating oxTMB, which can also be detected through UV-Vis spectrophotometry or the RGB channels (R/G/B) of a smartphone. The designed dual-mode biosensor features a simple structure, is easy to use, and has high specificity and selectivity. In the electrochemical mode, this sensor can detect OTA in the range of 1 fg/mL to 250 ng/mL, with a limit of detection (LOD) of 0.22 fg/mL. For the colorimetric detection mode, the LOD is 0.25 fg/mL, with a range of 1 fg/mL to 100 ng/mL. For the RGB mode, the LOD is 0.4 fg/mL. Using this biosensor, food extracts from corn and wheat samples, as well as diluted red wine samples, were tested, yielding results consistent with those from commercial enzyme-linked immunosorbent assay kits.
Literature information /Literature information
LI Y-L, CHEN Y-Y, XIE F-T, et al. Smartphone-based dual-mode aptasensor with bifunctional metal-organic frameworks as signal probes for ochratoxin A detection [J]. Food Chem, 2025, 464: 141540.
https://doi.org/10.1016/j.foodchem.2024.141540
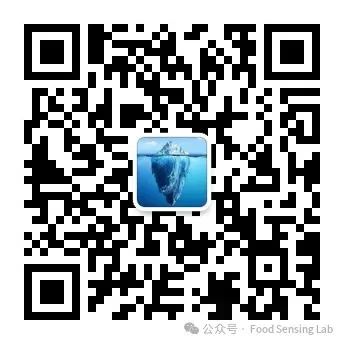
Scan the QR code above
Follow us
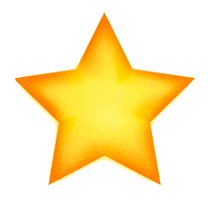
END
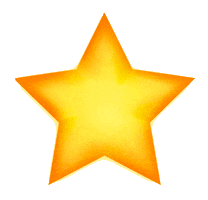
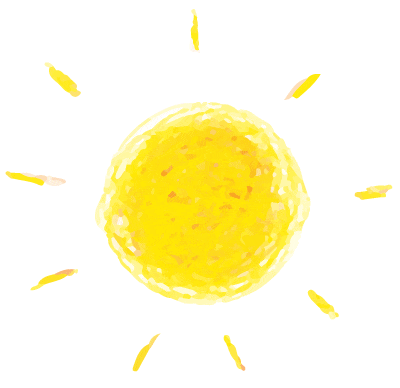
For submissions, recommendations, and collaborations, please contact the email
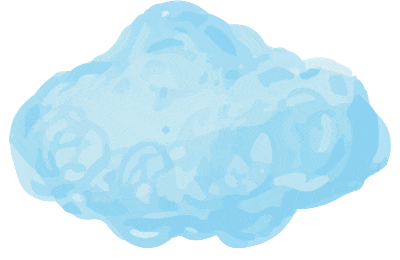
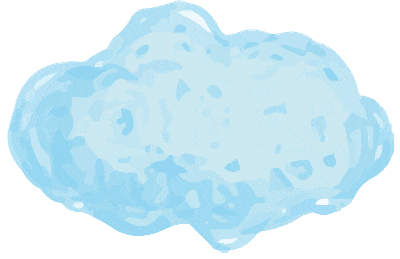
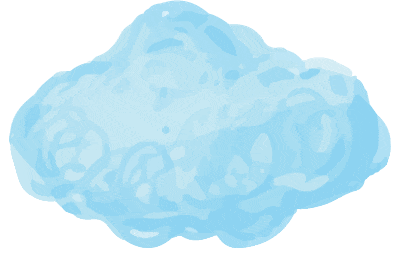
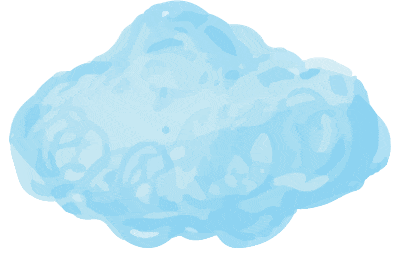
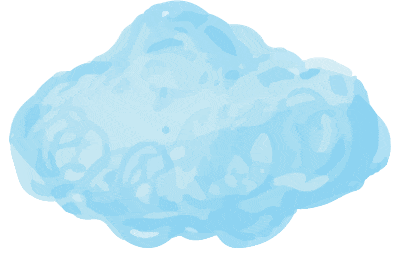