One of the outstanding features of the Da Vinci is that it performs well academically. (In 2022, there were 3,000 papers on Da Vinci globally, totaling over 35,000.)MedRobot will gradually release more paper content to help doctors find ideas and write good papers.
Robotics is a highly forward-looking discipline. A reasonable plan for the future development of medical robotics cannot be separated from a clear understanding of the latest research achievements and their clinical needs and commercialization.
How to choose a topic for writing a paper on robotics? Today, MedRobot has distilled a high-scoring review from Pierre E. Dupont et al. at Harvard Medical School, published in “Science Robotics” (一区, IF=25), titled “A decade retrospective of medical robotics research from 2010 to 2020,” which retrieved the most cited and pioneering papers from 2010 to 2020, identifying 8 key research themes in the field of medical robotics and conducted in-depth analysis and summary.
More than 30 years ago, the first batch of experts began to explore the use of robotic arms in surgical operations.
20 years ago, the first commercial robotic system was installed in hospitals.
In the past decade, there has been a tremendous advancement in the field of medical robotics.
Currently, thousands of robotic surgical systems have been installed worldwide, with millions of surgeries performed. As the medical system increasingly accepts surgical robots, researchers in the robotics field are focusing more on what the next generation of medical robots will look like.
This article focuses on a retrospective assessment of the major achievements in medical robotics over the past 10 years.
# Hot Topics
First, the authors identified 8 hot topics by searching for papers on medical robotics published from 2010 to 2020 in the Web of Science, some of which are related to specific clinical applications (such as laparoscopic robots), while others are related to enabling technologies widely used in medicine (such as soft robotics)..
The following figure shows that the number of publications on medical robotics in engineering and medical journals has increased exponentially, from 6 papers in 1990 to over 3,500 in 2020.
Due to the success of Intuitive Surgical’s Da Vinci robot, medical papers mainly focus on laparoscopic robots, accounting for 60% to 70% of the total, with over 1,300 papers published in 2020.
In contrast, as this technology matured, the number of engineering papers on laparoscopy peaked at 126 papers in 2019.
Engineering papers focus mainly on therapeutic rehabilitation and assistive wearable robots. These two hot topics account for about 80% of the medical robotics papers published in engineering journals over the past decade. Although the number of publications on these two topics was equal in 2010, the publication volume of therapeutic rehabilitation robots significantly exceeded that of assistive wearable robots in the following 10 years. Notably, the number of medical papers on these topics is less than 25% of the number of engineering papers, which may be due to the fact that medical journal papers typically report the results of clinical trials, which take more time and cost than engineering research.
The technology shown in the following figure is far less mature than that shown in the above figure, resulting in fewer publications in both engineering and medical journals. Among them, magnetic drive technology is the most mature, with both engineering and medical papers showing exponential growth, with medical papers lagging behind engineering papers. The continued development of this topic depends, to a certain extent, on whether the clinical applications of microrobots can be fully developed.
Laparoscopic robots may be the most mature and commercially successful area of medical robotic technology.
Over the past decade, significant progress has been made clinically, commercially, and academically.
Most research on laparoscopic robots is clinical research aimed at comparing the efficacy of robotic versus manual laparoscopic techniques in various surgical procedures. For example, radical prostatectomy, radical cystectomy, rectal cancer resection, and hysterectomy.
From 2010 to 2020, the continuous evolution of Intuitive Surgical’s Da Vinci robot. The system can now install endoscopes and laparoscopic instruments on all robotic arms and semi-automatic arms, and has improved instrument coupling.
In the past decade, at least 50 different instruments have been designed for the Da Vinci robot. Moreover, according to Intuitive Surgical’s annual reports, the use of Da Vinci has grown rapidly, with over 1.2 million surgeries completed in 2019. At the same time, the patents that monopolize Intuitive Surgical’s position in robotic laparoscopic surgery have begun to expire, and several large medical device companies have started to develop their own robots.
Over the past decade, academic research has made progress in two areas.
The first is using laparoscopic robots as a platform to develop enhanced capabilities. This mainly includes the introduction of open-platform robots for research, preliminary development of surgical automation, and integration of force sensing into laparoscopic tools.
The second is new robotic architectures to reduce surgical invasiveness. Single-port surgical systems have received the most attention, such as the commercially launched Da Vinci SP by Intuitive Surgical.
▲Da Vinci SP
(Image source: Intuitive Surgical)
-
Open-platform endoscopic surgical robots
-
-
Navigation, intraoperative imaging, and visualization
-
Contact force sensing and control
-
Single-port endoscopic surgical robots
-
# Non-laparoscopic Procedure-Specific Robots
Inspired by the success of the Da Vinci robot in laparoscopic surgery, in the past decade, surgeons and engineers have also been exploring new robotic solutions for non-laparoscopic surgeries.
-
Endoluminal and natural orifice surgery
-
Several exciting developments will promote a new round of innovation in surgical-specific robotic platforms. In the past decade, research has been conducted on electrode array steering and cochlear implant placement. These examples illustrate the potential to create new deep navigation platforms using soft robotics and possible magnetic drives. Exciting work has also been done in combining manipulation and diagnostic sensing. Researchers believe that real-time sensing in vivo needs further study to improve surgical outcomes. Systems that combine intraoperative perception with adaptive assistive behaviors (virtual fixtures or shared control) will also help surgeons achieve rapid clinical deployment and improve perception and performance.
# Assistive Wearable Robotics
Assistive wearable robots focus on the design and control of their devices, aiming to improve the mobility or functionality of patients with musculoskeletal or neuromuscular injuries. Notable advances in this field include the development of powered prosthetics for upper and lower limb amputees, as well as exoskeletons for patients with neuromuscular injuries, such as spinal cord injuries, strokes, multiple sclerosis, or cerebral palsy. Although the history of this field dates back at least to the early 1960s, it was not until the decade from 2010 to 2020 that assistive wearable robots were fully realized.
Powered lower limb prostheses
Neurally controlled upper limb prostheses
Lower limb exoskeletons (LLEs)
▲UGO lower limb exoskeleton system developed by RoboCT
# Therapeutic Rehabilitation Robots
(Therapeutic Rehabilitation Robots)
Assistive exoskeletons and prosthetics aim to replace lost functions, while rehabilitation robots are designed to provide repetitive motion therapy for limbs after neurological injuries (most commonly stroke and spinal cord injury), thus restoring patients’ own abilities. Therapeutic assistive robotic devices can induce or promote neuroplasticity, performing reaching, grasping, walking, and ankle movements to restore range of motion and coordination, and restore patients’ limb function, enabling self-care, independent living, and even return to work in some cases without robotic support.
Since their introduction in the early 1990s, rehabilitation robots have made significant progress in design, manufacturing, control, and clinical translation as a means to provide precise, repetitive motion therapy. In the decade before 2010, major research achievements included clinical evaluations and commercialization of the first generation of robotic devices developed for neurorehabilitation, including exoskeletons for gait rehabilitation, such as Lokomat, and end-effector-type robots for upper limb rehabilitation, such as InMotion ARM. Since the initial development, researchers began to develop new exoskeleton-type robots for the upper limb in the early 21st century, targeting specific joint movements at the distal ends of the elbow and shoulder, while lower limb exoskeletons promote ground walking. From 2010 to 2020, researchers completed foundational work on control algorithms aimed at better coordinating movements between the robot and the patient.
Key areas include four aspects:
The first is the design of novel exoskeleton styles, focusing on the distal joints of the upper limb, incorporating compliance and soft materials in both drive and structure;
The second is the development of new control algorithms to regulate the interaction between the patient and the robot, maximizing patient engagement.
The third is the creation of intention detection methods to infer and support the desired actions of patients, rather than specifying or pre-programming the robot’s motion trajectory.
The fourth is the promotion of robotic devices for objective and quantitative assessment of neural recovery.
▲Robotic technology used for rehabilitation
(Image source: SelectMedical)
Future Prospects:
Future research will focus on better understanding the mechanisms of neuroplasticity, including how to reliably induce and utilize it to maximize therapeutic effects. This relies on advances in neuroscience, including new technologies for recording neuronal activity. Advances in robotic technology are also crucial to achieving the above goals, including developing more suitable devices and embedding more precise sensing and actuation in devices to determine the upper and lower limb distal degrees of freedom that can best facilitate functional and independence recovery. Finally, advanced control algorithms can more accurately characterize patients’ abilities in real time, not only adjusting the level of support required to complete actions but also applying appropriate resistance or challenges.
# Capsule Robots
At the beginning of the century, Given Imaging (now Medtronic) launched the wireless capsule endoscope as a minimally invasive method for examining the gastrointestinal tract. Just swallowing a “pill” can collect images deep within the intestines, a possibility that has revolutionized the field of gastroenterology and led to a whole new area of research: capsule medical robotics.
▲Medtronic Pillcam capsule robot
(Image source: SelectMedical)
Researchers quickly realized that traditional capsule endoscopes passively move through the gastrointestinal tract and are limited by the inability to interact with the intestines and intervene. The first natural solution to this problem was to adopt “onboard drive,” using internal micro-motion mechanisms (such as legs) to actively control the capsule. However, enthusiasm for this approach quickly waned: using existing technology to integrate complex mechanisms, including sufficient power supply, into a “pill-sized” device (typically 24 mm in length and 11 mm in diameter) is impractical.
To address this issue, researchers explored magnetic drive as an alternative method. The use of magnetic coupling bypasses the need for complex mechanisms, reducing onboard power requirements and thus lowering the overall size and complexity of the device. This form of actuation manipulates the capsule (containing embedded magnets) through an externally generated magnetic field. This simple mechanical arrangement allows for precise control of capsule orientation and induces relative motion. The magnetic field can be generated by permanent magnets or electromagnets, with electromagnets providing better control over the magnitude of the magnetic field, but producing a lower volume magnetic flux density than permanent magnets. Medical capsule robots are now a viable clinical alternative to standard interventional endoscopic examinations.
Future Prospects:
As the next decade begins, capsule robots will combine with intelligent magnetic control and multimodal imaging (such as multispectral, autofluorescence, and micro/nano robots) to provide unprecedented diagnostic and therapeutic capabilities. In addition to clinical uses, they can provide research platforms to delve deep into the human body and address other scientific issues related to the microbiome.
In the future, exciting progress may also be made in energy storage or wireless power transmission, revitalizing onboard drive methods or “multiscale operations,” such as deploying an army of interventional microrobots from one capsule robot. Regardless of the future, medical capsule robots remain an exciting, rapidly developing, and highly impactful research area.
# Magnetic Actuation for Medicine
(Magnetic Actuation for Medicine)
As early as before being used for in-body imaging, magnetic fields were used for surgery. Records of using magnetic fields to extract iron filings accidentally embedded in the eye date back at least to the 17th century and the Industrial Revolution. In the 1950s, the first studies on using magnetic fields to guide catheters equipped with magnets were conducted.
However, it was not until 2003 that Stereotaxis’s Niobe robotic magnetic navigation system was commercialized, which uses two moving permanent magnets to generate a changing magnetic field to guide catheters for cardiac ablation procedures (electrophysiological procedures). Despite the low market penetration of this magnetic-guided catheter system, interest from researchers and medical device companies has grown in the past decade, with a linear increase in the number of papers on the subject.
-
Modeling multi-DOF electromagnetic navigation systems
-
Magnetically guided microrobots
-
Magnetic locomotion strategies at millimeter scales
-
Magnetically guided catheters
▲(A)Traditional laparoscopic surgery; (B)Locally magnetic-driven robotic laparoscopic surgery
(Image source: Robotics at Leeds)
# Soft Robotics for Medicine
Soft robotics are essentially compliant structures and smart materials, closely related to biomimetics and bioinspired designs from the outset. On the other hand, the growing interest in soft biomimetic robots has also promoted research on smart materials, which can range from macroscopic to nanoscale, for fabricating soft robots or providing them with sensing and actuation capabilities. For example, most research on artificial skin with sensing capabilities can be found in the literature on soft robots and devices.
Over the past decade, there have been many foundational reviews or survey papers on various applications of soft biomimetic robots, as well as many research papers and reviews on new smart materials, in which traditional silicon-based sensing technologies have been replaced by silicon-based technologies with intelligent behaviors.
In terms of the most highly cited papers over the past decade, excluding material research papers and survey papers, soft robotics can be divided into two categories: one is wearable soft robots for rehabilitation or human enhancement. The second includes interventional and surgical robots or related components.
Three main themes in the interventional and surgical fields are:
(1) Soft devices for surgery or intervention, where entire traditional devices are replaced by soft robotic designs at macro and micro scales;
(2) Soft, biomimetic, or compliant components that work as independent devices or are integrated into traditional systems;
(3) Soft components and systems for advanced simulators for training and research on specific physiological functions between robots and biological artificial organs.
Although the soft robotics field has yet to produce representative examples, it is guiding the design and development of most medical devices. Meanwhile, soft robotics technology is also promoting research on soft materials and new manufacturing techniques, opening up unexpected pathways in biomedical applications.
▲Soft robots for surgery and drug delivery
(Image source: Nature Reviews)
# Continuum Robots for Medicine
Continuum robots change shape by bending and deforming without discrete joints, allowing for 3D deformation, which enables these robots to perform surgery through smaller channels compared to traditional robotic architectures. They can enter the human body through natural openings, navigate through internal cavities, and bypass critical structures while traversing solid tissues. The bending compliance of continuum robots also enhances their safety compared to traditional designs.
In the decade before 2010, major research advancements involved developing design principles and mechanics-based kinematic models for tendon- and multi-skeleton-driven continuum robotic structures. This facilitated the commercialization of medical robots, such as tendon-driven cardiac catheters from Hansen Medical.
Additionally, a tendon-driven design was proposed, replacing the flexible backbone with a series of short cylindrical links connected by spherical joints. This design became the basis for Medrobotics’ commercial surgical robots. In the 2000s, the concept of concentric tube robots was first introduced, but a complete description of the design principles and kinematic models for this architecture was not completed until 2010.
From 2010 to 2020, research on continuum robots primarily focused on four areas:
-
Integrating external contact and loads into robotic modeling and control
-
Developing methods to control the stiffness of robots
-
Creating soft continuum robots
-
Designing continuum robots for specific clinical applications.
-
Extending kinematic models to consider external contacts and loads
-
-
-
Application-specific continuum robot design
▲Classification of continuum robots based on their structure and driving methods
(Image source: Hansen Medical)
# Discussion
Further advancement of medical robotics relies on a deep understanding of how robots and their underlying technologies add value to medicine. Although in almost every other industry, it is widely believed that robots as autonomous entities reduce labor costs, medical robots, at least so far, have brought added value in other applications.
In rehabilitation therapy, it can be said that the current added value lies in providing more repetitions rather than improving the quality of repetitions; Energy delivery robots used for radiation therapy provide a combination of precision, repeatability, and speed that is difficult to match by other means; Compared to non-robotic devices, powered prosthetics can directly improve patients’ prognosis by expanding the number and quality of daily living tasks; Capsule robots may ultimately replace some open intestinal surgeries, improving diagnoses in hard-to-reach areas of the body and reducing the discomfort of existing endoluminal intestinal surgeries.
In guiding robotic technology research to maximize value addition, the most important technical goal is to achieve new interventions that cannot be realized or are impractical based on existing technologies. In the next decade, soft robotics is likely to become a very important enabling technology. Most of the most promising work is currently in the materials field, involving the manufacture of thin polymer layers with embedded sensors and actuators. Although this work may seem far from medical applications, these capabilities could have a huge impact on interventional, rehabilitation, and assistive robotics. Other enabling technologies such as sensing, imaging, actuation, and energy storage may bring leapfrog developments to consumer electronics.
A technology that can not only give rise to a new type of surgical procedure but also add value to medical robots is also of extraordinary significance. The combination of preoperative and intraoperative imaging with flexible, ergonomic enhanced surgical tools is a good example. The value of this approach may continue to grow in the future. Translating laboratory cellular and molecular imaging into in situ surgery will provide improved tissue detection, labeling, and targeting for both macroscopic and cellular-level treatments, further expanding the functional capabilities of surgical interventions. Simplifying intraoperative decision-making and optimization, and achieving higher consistency and accuracy to avoid potential postoperative complications, could fundamentally change surgical path planning.
Another way for robots to add value is through increased autonomy. The cutting edge of autonomy in medical robots lies in giving robots the ability to formulate and change their plans and movements based on real-time sensor data. For example, autonomous laparoscopic surgical removal of cancerous lesions or autonomous catheter-based heart valve repair. This level of autonomy not only poses technical challenges but also raises regulatory, ethical, and legal issues that need to be addressed, and will increase commercialization costs. Therefore, gradually increasing this autonomous function in existing medical robots will be much easier, and the value of these robots can be demonstrated without considering autonomous functions. For example, automatic suturing in laparoscopic surgery, autonomous navigation of flexible endoscopes, or autonomous electrophysiological catheter mapping in the heart.
The evolutionary trend of gradual autonomy (as shown in the figure below) will provide time for the development of necessary technologies such as algorithms and sensors while giving stakeholders time to gradually build appropriate regulatory and legal frameworks. In the short term, commercializing medical robots that must have autonomous capabilities will be more challenging, but in the long term, they may have the highest value.
▲Specific application trends for increasing autonomy in medical robots
Of the more than 19,000 engineering papers published on medical robotics since 1990, only a handful can empower existing commercial medical robots. Even highly influential papers with high technical impact have few patent citations. To some extent, this may be due to the huge lag between technological development and its commercial application. The mismatch between technological research and the realities of medical device commercialization is also a contributing factor.
Applying robotic technology to clinical settings requires more than just cited research articles; it is essential to identify genuine clinical needs, develop relevant technologies to meet those clinical needs, and consider the details of how robots can add value to clinicians and patients. The development of technology must also fully consider the administrative and financial constraints of hospitals, not to hinder the improvement of clinical workflows; potential risks must be identified early to obtain ethical approval; and attractive business models must be developed to ensure sufficient investment to navigate the complex path to commercial success that medical devices must go through. To maximize the chances of success, technology researchers must step out of the ivory tower and establish deep collaborations with clinicians, regulatory bodies, investors, and the business community.
Editor-in-Chief|Qing Zhao Review|Yi He Typesetting|Miya
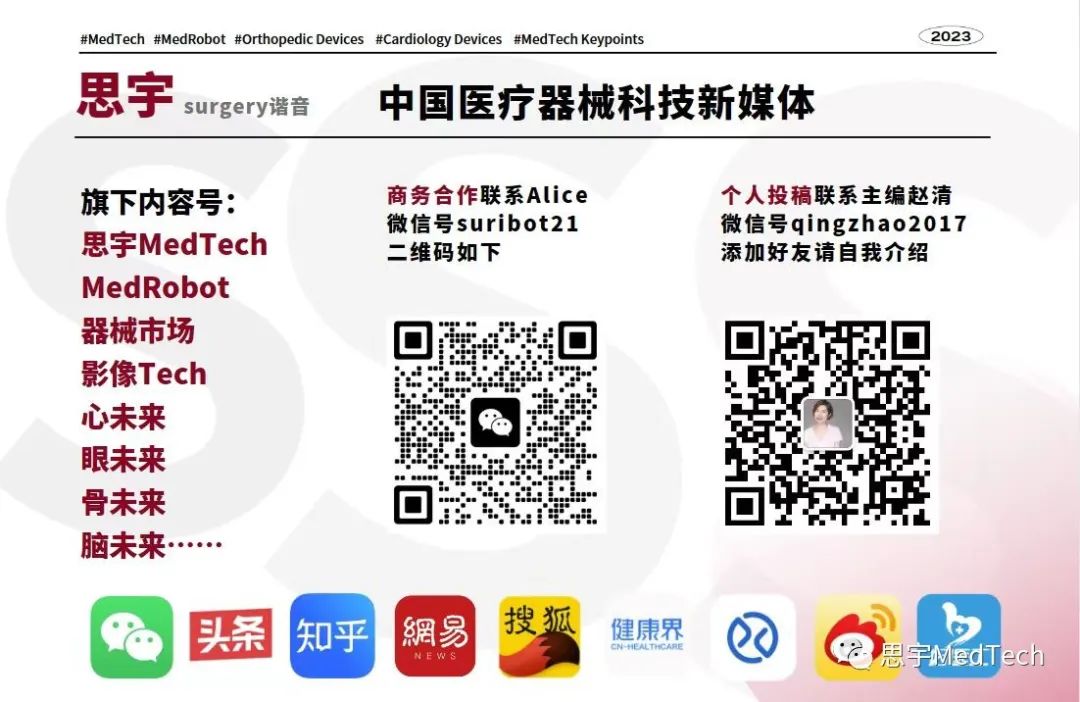